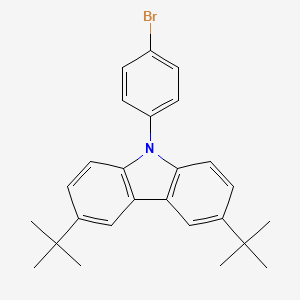
9-(4-Bromophenyl)-3,6-DI-tert-butyl-9H-carbazole
Overview
Description
9-(4-Bromophenyl)-3,6-Di-tert-butyl-9H-carbazole is a complex organic compound characterized by its bromophenyl group and carbazole core structure
Preparation Methods
Types of Reactions:
Oxidation: The compound can undergo oxidation reactions to form various oxidized derivatives.
Reduction: Reduction reactions can be used to modify the electronic properties of the compound.
Substitution: Substitution reactions, particularly at the bromophenyl group, are common and can introduce different functional groups.
Common Reagents and Conditions:
Oxidation: Common oxidizing agents include potassium permanganate (KMnO4) and chromic acid (H2CrO4).
Reduction: Reducing agents such as lithium aluminum hydride (LiAlH4) and hydrogen gas (H2) are used.
Substitution: Nucleophilic substitution reactions often use strong bases like sodium hydride (NaH) and polar aprotic solvents.
Major Products Formed:
Oxidation Products: Various carbonyl-containing derivatives.
Reduction Products: Reduced forms with altered electronic properties.
Substitution Products: Derivatives with different substituents at the bromophenyl position.
Scientific Research Applications
Chemistry: In chemistry, 9-(4-Bromophenyl)-3,6-Di-tert-butyl-9H-carbazole is used as an intermediate in the synthesis of more complex organic molecules. Its bromophenyl group makes it a versatile building block for constructing larger molecular frameworks.
Medicine: In the medical field, derivatives of this compound are being explored for their potential use in pharmaceuticals. Its ability to interact with biological targets makes it a candidate for drug development.
Mechanism of Action
The mechanism by which 9-(4-Bromophenyl)-3,6-Di-tert-butyl-9H-carbazole exerts its effects depends on its specific application. In OLEDs, for example, the compound acts as an electron transport material, facilitating the movement of electrons within the device. The molecular targets and pathways involved include the interaction with other organic materials in the device, leading to efficient light emission.
Comparison with Similar Compounds
9-(4-Methoxyphenyl)-3,6-Di-tert-butyl-9H-carbazole: Similar structure but with a methoxy group instead of bromophenyl.
9-(4-Nitrophenyl)-3,6-Di-tert-butyl-9H-carbazole: Similar structure but with a nitro group instead of bromophenyl.
9-(4-Chlorophenyl)-3,6-Di-tert-butyl-9H-carbazole: Similar structure but with a chlorophenyl group instead of bromophenyl.
Uniqueness: 9-(4-Bromophenyl)-3,6-Di-tert-butyl-9H-carbazole is unique due to its bromophenyl group, which imparts different electronic and chemical properties compared to other similar compounds. This makes it particularly useful in specific applications where the bromine atom plays a critical role.
Properties
IUPAC Name |
9-(4-bromophenyl)-3,6-ditert-butylcarbazole | |
---|---|---|
Source | PubChem | |
URL | https://pubchem.ncbi.nlm.nih.gov | |
Description | Data deposited in or computed by PubChem | |
InChI |
InChI=1S/C26H28BrN/c1-25(2,3)17-7-13-23-21(15-17)22-16-18(26(4,5)6)8-14-24(22)28(23)20-11-9-19(27)10-12-20/h7-16H,1-6H3 | |
Source | PubChem | |
URL | https://pubchem.ncbi.nlm.nih.gov | |
Description | Data deposited in or computed by PubChem | |
InChI Key |
LAHSPJHDQUQBTB-UHFFFAOYSA-N | |
Source | PubChem | |
URL | https://pubchem.ncbi.nlm.nih.gov | |
Description | Data deposited in or computed by PubChem | |
Canonical SMILES |
CC(C)(C)C1=CC2=C(C=C1)N(C3=C2C=C(C=C3)C(C)(C)C)C4=CC=C(C=C4)Br | |
Source | PubChem | |
URL | https://pubchem.ncbi.nlm.nih.gov | |
Description | Data deposited in or computed by PubChem | |
Molecular Formula |
C26H28BrN | |
Source | PubChem | |
URL | https://pubchem.ncbi.nlm.nih.gov | |
Description | Data deposited in or computed by PubChem | |
DSSTOX Substance ID |
DTXSID50727295 | |
Record name | 9-(4-Bromophenyl)-3,6-di-tert-butyl-9H-carbazole | |
Source | EPA DSSTox | |
URL | https://comptox.epa.gov/dashboard/DTXSID50727295 | |
Description | DSSTox provides a high quality public chemistry resource for supporting improved predictive toxicology. | |
Molecular Weight |
434.4 g/mol | |
Source | PubChem | |
URL | https://pubchem.ncbi.nlm.nih.gov | |
Description | Data deposited in or computed by PubChem | |
CAS No. |
601454-33-5 | |
Record name | 9-(4-Bromophenyl)-3,6-di-tert-butyl-9H-carbazole | |
Source | EPA DSSTox | |
URL | https://comptox.epa.gov/dashboard/DTXSID50727295 | |
Description | DSSTox provides a high quality public chemistry resource for supporting improved predictive toxicology. | |
Record name | (9-(4-Bromophenyl))-3,6-di-tert-butyyl-9H-carbazole | |
Source | European Chemicals Agency (ECHA) | |
URL | https://echa.europa.eu/information-on-chemicals | |
Description | The European Chemicals Agency (ECHA) is an agency of the European Union which is the driving force among regulatory authorities in implementing the EU's groundbreaking chemicals legislation for the benefit of human health and the environment as well as for innovation and competitiveness. | |
Explanation | Use of the information, documents and data from the ECHA website is subject to the terms and conditions of this Legal Notice, and subject to other binding limitations provided for under applicable law, the information, documents and data made available on the ECHA website may be reproduced, distributed and/or used, totally or in part, for non-commercial purposes provided that ECHA is acknowledged as the source: "Source: European Chemicals Agency, http://echa.europa.eu/". Such acknowledgement must be included in each copy of the material. ECHA permits and encourages organisations and individuals to create links to the ECHA website under the following cumulative conditions: Links can only be made to webpages that provide a link to the Legal Notice page. | |
Retrosynthesis Analysis
AI-Powered Synthesis Planning: Our tool employs the Template_relevance Pistachio, Template_relevance Bkms_metabolic, Template_relevance Pistachio_ringbreaker, Template_relevance Reaxys, Template_relevance Reaxys_biocatalysis model, leveraging a vast database of chemical reactions to predict feasible synthetic routes.
One-Step Synthesis Focus: Specifically designed for one-step synthesis, it provides concise and direct routes for your target compounds, streamlining the synthesis process.
Accurate Predictions: Utilizing the extensive PISTACHIO, BKMS_METABOLIC, PISTACHIO_RINGBREAKER, REAXYS, REAXYS_BIOCATALYSIS database, our tool offers high-accuracy predictions, reflecting the latest in chemical research and data.
Strategy Settings
Precursor scoring | Relevance Heuristic |
---|---|
Min. plausibility | 0.01 |
Model | Template_relevance |
Template Set | Pistachio/Bkms_metabolic/Pistachio_ringbreaker/Reaxys/Reaxys_biocatalysis |
Top-N result to add to graph | 6 |
Feasible Synthetic Routes
Disclaimer and Information on In-Vitro Research Products
Please be aware that all articles and product information presented on BenchChem are intended solely for informational purposes. The products available for purchase on BenchChem are specifically designed for in-vitro studies, which are conducted outside of living organisms. In-vitro studies, derived from the Latin term "in glass," involve experiments performed in controlled laboratory settings using cells or tissues. It is important to note that these products are not categorized as medicines or drugs, and they have not received approval from the FDA for the prevention, treatment, or cure of any medical condition, ailment, or disease. We must emphasize that any form of bodily introduction of these products into humans or animals is strictly prohibited by law. It is essential to adhere to these guidelines to ensure compliance with legal and ethical standards in research and experimentation.