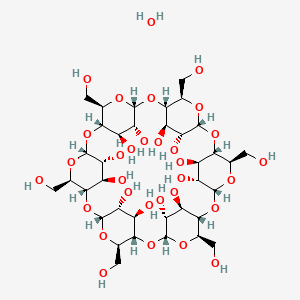
alpha-Cyclodextrin hydrate
Overview
Description
?-Cyclodextrin (hydrate) is a cyclic oligosaccharide composed of glucose subunits linked by α-1,4 glycosidic bonds. It is part of the cyclodextrin family, which includes α-cyclodextrin, β-cyclodextrin, and γ-cyclodextrin, each differing in the number of glucose units. Cyclodextrins are produced from starch by enzymatic conversion and are used in various industries, including food, pharmaceuticals, and chemical industries .
Preparation Methods
Synthetic Routes and Reaction Conditions
?-Cyclodextrin (hydrate) is typically synthesized by treating starch with specific enzymes such as cyclodextrin glycosyltransferase. The enzymatic conversion involves the cleavage of starch into smaller glucose units, which then cyclize to form the cyclic structure of cyclodextrins .
Industrial Production Methods
Industrial production of ?-Cyclodextrin (hydrate) involves large-scale enzymatic processes where starch is treated with cyclodextrin glycosyltransferase. The resulting mixture is then purified to isolate the desired cyclodextrin. This process is optimized to produce high yields of cyclodextrins with minimal by-products .
Chemical Reactions Analysis
Types of Reactions
?-Cyclodextrin (hydrate) undergoes various chemical reactions, including:
Reduction: Involves the removal of oxygen atoms or the addition of hydrogen atoms.
Substitution: Involves the replacement of one functional group with another.
Common Reagents and Conditions
Common reagents used in these reactions include oxidizing agents like hydrogen peroxide, reducing agents like sodium borohydride, and various substituting agents depending on the desired modification. Reaction conditions typically involve controlled temperatures and pH levels to ensure the stability of the cyclodextrin structure .
Major Products Formed
The major products formed from these reactions include modified cyclodextrins with altered solubility, stability, and inclusion properties. These modifications enhance the utility of cyclodextrins in various applications .
Scientific Research Applications
Food Technology
Nutritional and Technological Properties
α-Cyclodextrin is primarily utilized as a food ingredient due to its ability to modify flavor profiles, stabilize emulsions, and act as a carrier for vitamins and flavors. Its dietary fiber-like properties contribute to increased fecal bulk and modulation of glycemic response, making it suitable for functional food formulations.
Proposed Uses in Food Products:
- Bakery Products: Enhances texture and moisture retention.
- Dairy Products: Acts as a stabilizer in yogurt and cheese.
- Beverages: Improves flavor stability in juices and soft drinks.
Case Study: Regulatory Approval
A comprehensive assessment by Food Standards Australia New Zealand (FSANZ) concluded that α-cyclodextrin can be safely used as a novel food ingredient without adversely affecting the absorption of fat-soluble nutrients .
Application Area | Functionality | Examples |
---|---|---|
Bakery Products | Moisture retention, texture enhancement | Breads, pastries |
Dairy Products | Stabilization of emulsions | Yogurt, cheese |
Beverages | Flavor stability | Juices, soft drinks |
Pharmaceutical Applications
Drug Delivery Systems
α-Cyclodextrin is extensively studied for its role in enhancing the solubility and bioavailability of poorly soluble drugs. Its ability to form inclusion complexes allows for improved drug formulation.
Key Benefits:
- Increased Solubility: Enhances the dissolution rate of hydrophobic drugs.
- Controlled Release: Facilitates sustained release profiles.
Case Study: Inclusion Complexes
Research demonstrated that α-CD can effectively encapsulate various pharmaceutical compounds, leading to improved therapeutic efficacy . For instance, studies on the inclusion of anti-inflammatory drugs showed significant enhancement in their solubility and stability.
Drug Type | Encapsulation Efficiency | Release Profile |
---|---|---|
Anti-inflammatory | High | Sustained |
Anticancer | Moderate | Controlled |
Antibiotics | High | Immediate |
Environmental Applications
Ethylene Scavengers
Recent advancements have highlighted the potential of α-cyclodextrin nanosponges as ethylene scavengers in post-harvest fruit preservation. The porous structure of these nanosponges allows for effective ethylene absorption, extending the shelf life of climacteric fruits.
Case Study: Scaling Up Production
A study focused on scaling up α-cyclodextrin nanosponges demonstrated their effectiveness in absorbing ethylene at rates beneficial for fruit storage . The results indicated that these nanosponges could significantly reduce ethylene levels in storage environments.
Application | Ethylene Absorption Rate | Impact on Shelf Life |
---|---|---|
Climacteric Fruits | 93 µL/kg/h | Extended by several days |
Structural Studies
Molecular Dynamics and Hydration Effects
Understanding the structural properties of α-cyclodextrin is crucial for optimizing its applications. Recent studies utilizing molecular dynamics simulations have revealed insights into the hydration states that affect its inclusion capacity .
Key Findings:
- Different hydration states significantly influence the guest inclusion properties.
- The formation of hydrates can be controlled through specific preparation methods.
Mechanism of Action
?-Cyclodextrin (hydrate) exerts its effects through the formation of inclusion complexes with guest molecules. The hydrophobic cavity of the cyclodextrin traps hydrophobic molecules, while the hydrophilic exterior interacts with the aqueous environment. This mechanism enhances the solubility and stability of the guest molecules, facilitating their delivery and controlled release .
Comparison with Similar Compounds
Similar Compounds
α-Cyclodextrin: Composed of six glucose units.
β-Cyclodextrin: Composed of seven glucose units.
γ-Cyclodextrin: Composed of eight glucose units.
Uniqueness
?-Cyclodextrin (hydrate) is unique due to its specific number of glucose units, which determines its cavity size and inclusion properties. This makes it suitable for encapsulating molecules of specific sizes and enhancing their solubility and stability .
Biological Activity
Alpha-cyclodextrin (α-CD) is a cyclic oligosaccharide composed of six glucose units. It has garnered significant interest in the fields of pharmacology, nutrition, and biotechnology due to its unique properties, including its ability to form inclusion complexes with various compounds. This article explores the biological activity of α-CD hydrate, focusing on its effects on cellular hydration, longevity, and potential therapeutic applications.
Water Transport and Cellular Hydration
Recent studies have demonstrated that α-CD enhances cellular water uptake through aquaporins, which are channel proteins that facilitate water transport across cell membranes. In a study using Xenopus laevis oocytes expressing human aquaporin 1 (AQP1), α-CD was found to significantly improve water permeability, suggesting a mechanism by which it can enhance cell hydration . This effect is attributed to the interaction of α-CD with membrane lipids, particularly phospholipids enriched with unsaturated fatty acids, which promote aquaporin functionality .
Longevity Effects
In addition to its role in hydration, α-CD has been shown to promote longevity in model organisms such as the nematode Caenorhabditis elegans. In experiments where nematodes were treated with low concentrations (0.05-0.5% w/v) of α-CD, researchers observed an increase in lifespan and vitality during mid-life stages . This suggests that α-CD may have broader implications for aging and age-related diseases.
SARS-CoV-2 Defense
A pilot study investigated the potential of a food supplement combining α-CD and hydroxytyrosol to enhance defenses against SARS-CoV-2. The results indicated that participants who consumed this supplement did not test positive for the virus over a 15-day period, despite being at higher risk. Furthermore, in cases where subjects tested positive, those using the supplement showed a quicker reduction in viral load compared to controls . The mechanism is thought to involve the depletion of sphingolipids from cellular membranes by α-CD, potentially hindering viral entry into cells .
Case Studies and Research Findings
Study | Model Organism | Findings | Concentration |
---|---|---|---|
Study 1 | Xenopus laevis | Enhanced water uptake via aquaporins | 0.05-0.5% w/v |
Study 2 | C. elegans | Increased lifespan and vitality | 0.05-0.5% w/v |
Study 3 | Human volunteers | Reduced SARS-CoV-2 positivity rate | Supplementation |
Q & A
Basic Research Questions
Q. How can the structural properties of α-cyclodextrin hydrate be experimentally characterized?
- Methodological Answer : Structural characterization typically involves X-ray diffraction (XRD) to confirm crystallinity and cavity dimensions (6–7 Å diameter), nuclear magnetic resonance (NMR) to analyze glucopyranose unit connectivity, and polarimetry to measure specific optical rotation (+150.5° at 25°C in water). Thermogravimetric analysis (TGA) can assess hydrate stability, while dynamic light scattering (DLS) evaluates aggregation behavior in aqueous solutions .
Q. What experimental methods are used to determine the solubility of α-cyclodextrin hydrate in polar solvents?
- Methodological Answer : Solubility is quantified via gravimetric analysis by dissolving α-cyclodextrin in water under controlled agitation (25°C, 145 g/L solubility). For low-solubility solvents (e.g., methanol, ethanol), saturation is measured using UV-Vis spectroscopy or HPLC after filtration. Note that solubility decreases with temperature due to reduced hydrogen-bonding capacity .
Q. How are inclusion complexes of α-cyclodextrin hydrate prepared for drug delivery studies?
- Methodological Answer : Common methods include co-precipitation (mixing guest molecules with α-cyclodextrin in water, followed by lyophilization) and solvent evaporation (dissolving both components in a volatile solvent, then evaporating to form complexes). Characterization via phase solubility diagrams (Higuchi method) and fluorescence spectroscopy validates encapsulation efficiency .
Q. What analytical techniques confirm the formation of host-guest complexes with α-cyclodextrin?
- Methodological Answer : Fluorescence spectroscopy detects enhanced fluorescence intensity upon guest encapsulation (e.g., hydrophobic dyes). Isothermal titration calorimetry (ITC) quantifies binding constants, while HPLC monitors retention time shifts. Nuclear Overhauser effect (NOE) NMR identifies spatial interactions between host and guest .
Advanced Research Questions
Q. How can enzymatic synthesis of α-cyclodextrin be optimized using cyclodextrin glucosyltransferase (CGTase)?
- Methodological Answer : Optimize substrate-to-enzyme ratios (e.g., 400 units CGTase per gram starch) and reaction conditions (pH 8–9, 50–60°C). Use Bacillus macerans CGTase for higher α-cyclodextrin yield. Monitor reaction kinetics via HPLC and adjust ethanol concentrations to favor α-cyclodextrin over β/γ-forms .
Q. How do contradictions in hydration studies involving α-cyclodextrin arise, and how should they be resolved?
- Methodological Answer : Discrepancies in hydration metrics (e.g., phase angle vs. urine-specific gravity) stem from varied assessment methodologies . Use multimodal approaches : bioelectrical impedance analysis (BIA) for intracellular fluid changes, deuterium oxide (D₂O) tracer techniques for fluid retention, and in vitro aquaporin permeability assays (e.g., Xenopus oocyte models) to isolate molecular mechanisms .
Q. What experimental strategies elucidate α-cyclodextrin's interaction with cell membranes and aquaporins?
- Methodological Answer : Employ membrane fluidity assays (e.g., fluorescence polarization with diphenylhexatriene probes) and surface plasmon resonance (SPR) to study lipid-cyclodextrin binding. For aquaporin studies, use heterologous expression systems (e.g., human AQP1 in oocytes) with osmotic challenge tests to measure water permeability changes .
Q. How can α-cyclodextrin enhance the stability of labile compounds in pharmaceutical formulations?
- Methodological Answer : Design accelerated stability studies under stress conditions (heat, light, humidity) comparing free vs. complexed drugs. Use Fourier-transform infrared spectroscopy (FTIR) to detect chemical degradation and differential scanning calorimetry (DSC) to monitor phase transitions. For biologics, assess aggregation via size-exclusion chromatography (SEC) .
Q. What advanced methods validate α-cyclodextrin's role in hydrogel formation for controlled drug release?
- Methodological Answer : Synthesize hydrogels via physical crosslinking (e.g., Pluronic F-127/α-cyclodextrin systems). Characterize rheological properties using oscillatory rheometry and pore structure via cryo-SEM . Monitor drug release kinetics in simulated physiological buffers and model data using Higuchi or Korsmeyer-Peppas equations .
Q. How do computational models predict α-cyclodextrin's binding affinity for novel drug candidates?
Properties
IUPAC Name |
(1S,3R,5R,6S,8R,10R,11S,13R,15R,16S,18R,20R,21S,23R,25R,26S,28R,30R,31R,32R,33R,34R,35R,36R,37R,38R,39R,40R,41R,42R)-5,10,15,20,25,30-hexakis(hydroxymethyl)-2,4,7,9,12,14,17,19,22,24,27,29-dodecaoxaheptacyclo[26.2.2.23,6.28,11.213,16.218,21.223,26]dotetracontane-31,32,33,34,35,36,37,38,39,40,41,42-dodecol;hydrate | |
---|---|---|
Source | PubChem | |
URL | https://pubchem.ncbi.nlm.nih.gov | |
Description | Data deposited in or computed by PubChem | |
InChI |
InChI=1S/C36H60O30.H2O/c37-1-7-25-13(43)19(49)31(55-7)62-26-8(2-38)57-33(21(51)15(26)45)64-28-10(4-40)59-35(23(53)17(28)47)66-30-12(6-42)60-36(24(54)18(30)48)65-29-11(5-41)58-34(22(52)16(29)46)63-27-9(3-39)56-32(61-25)20(50)14(27)44;/h7-54H,1-6H2;1H2/t7-,8-,9-,10-,11-,12-,13-,14-,15-,16-,17-,18-,19-,20-,21-,22-,23-,24-,25-,26-,27-,28-,29-,30-,31-,32-,33-,34-,35-,36-;/m1./s1 | |
Source | PubChem | |
URL | https://pubchem.ncbi.nlm.nih.gov | |
Description | Data deposited in or computed by PubChem | |
InChI Key |
UQIQLAUJPPOBJR-HAPKRNSXSA-N | |
Source | PubChem | |
URL | https://pubchem.ncbi.nlm.nih.gov | |
Description | Data deposited in or computed by PubChem | |
Canonical SMILES |
C(C1C2C(C(C(O1)OC3C(OC(C(C3O)O)OC4C(OC(C(C4O)O)OC5C(OC(C(C5O)O)OC6C(OC(C(C6O)O)OC7C(OC(O2)C(C7O)O)CO)CO)CO)CO)CO)O)O)O.O | |
Source | PubChem | |
URL | https://pubchem.ncbi.nlm.nih.gov | |
Description | Data deposited in or computed by PubChem | |
Isomeric SMILES |
C([C@@H]1[C@@H]2[C@@H]([C@H]([C@H](O1)O[C@@H]3[C@H](O[C@@H]([C@@H]([C@H]3O)O)O[C@@H]4[C@H](O[C@@H]([C@@H]([C@H]4O)O)O[C@@H]5[C@H](O[C@@H]([C@@H]([C@H]5O)O)O[C@@H]6[C@H](O[C@@H]([C@@H]([C@H]6O)O)O[C@@H]7[C@H](O[C@H](O2)[C@@H]([C@H]7O)O)CO)CO)CO)CO)CO)O)O)O.O | |
Source | PubChem | |
URL | https://pubchem.ncbi.nlm.nih.gov | |
Description | Data deposited in or computed by PubChem | |
Molecular Formula |
C36H62O31 | |
Source | PubChem | |
URL | https://pubchem.ncbi.nlm.nih.gov | |
Description | Data deposited in or computed by PubChem | |
Molecular Weight |
990.9 g/mol | |
Source | PubChem | |
URL | https://pubchem.ncbi.nlm.nih.gov | |
Description | Data deposited in or computed by PubChem | |
Disclaimer and Information on In-Vitro Research Products
Please be aware that all articles and product information presented on BenchChem are intended solely for informational purposes. The products available for purchase on BenchChem are specifically designed for in-vitro studies, which are conducted outside of living organisms. In-vitro studies, derived from the Latin term "in glass," involve experiments performed in controlled laboratory settings using cells or tissues. It is important to note that these products are not categorized as medicines or drugs, and they have not received approval from the FDA for the prevention, treatment, or cure of any medical condition, ailment, or disease. We must emphasize that any form of bodily introduction of these products into humans or animals is strictly prohibited by law. It is essential to adhere to these guidelines to ensure compliance with legal and ethical standards in research and experimentation.