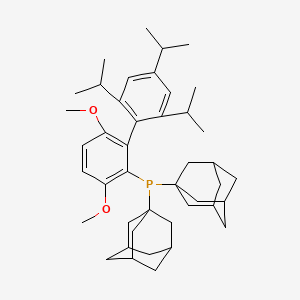
AdBrettPhos
Overview
Description
AdBrettPhos is a phosphine-based ligand used in the direct monoarylation of ammonia and suppresses diarylation . It is also a good ligand for five-membered heteroarene couplings and is employed in the preparation of phosphine-ligated palladium complexes to facilitate C-N coupling reactions .
Synthesis Analysis
This compound was synthesized and used along with tBuBrettPhos in C-O coupling reactions at low temperatures . Pd catalysts containing these ligands were used to couple electron-neutral aryl bromides with phenols at room temperature for the first time .Molecular Structure Analysis
The molecular formula of this compound is C43H61O2P . The average mass is 640.917 Da and the monoisotopic mass is 640.440918 Da .Chemical Reactions Analysis
This compound is used in the direct monoarylation of ammonia and suppresses diarylation . It is also used in five-membered heteroarene couplings and in the preparation of phosphine-ligated palladium complexes to facilitate C-N coupling reactions .Physical And Chemical Properties Analysis
This compound has a molecular formula of CHOP, an average mass of 640.917 Da, and a monoisotopic mass of 640.440918 Da . It has 2 H bond acceptors, 0 H bond donors, 9 freely rotating bonds, and 2 Rule of 5 violations . Its ACD/LogP is 13.59 .Scientific Research Applications
Catalyst System Improvement for Fluorination
AdBrettPhos has been identified as a key component in improving catalyst systems for the palladium-catalyzed fluorination of aryl triflates, a process relevant to the synthesis of biologically active compounds. The stable Pd(0) species [(1,5-cyclooctadiene)(L·Pd)2] (L = this compound) offers significant advancements, activating at room temperature under neutral conditions and leading to cleaner reaction profiles (Lee, Milner, & Buchwald, 2013).
Mechanism of Action
Target of Action
It is known to be a phosphine ligand , which suggests that it likely interacts with metal ions in its target molecules.
Mode of Action
As a phosphine ligand, AdBrettPhos can form complexes with metal ions, aiding in metal-catalyzed reactions . The bulky di(1-adamantyl)phosphino fragment in its structure may provide steric hindrance, influencing the geometry and reactivity of the metal center in these complexes .
Result of Action
This compound primarily aids in metal-catalyzed reactions, leading to the formation of bioactive molecules . The exact molecular and cellular effects would depend on the specific reactions it is involved in.
Action Environment
The action, efficacy, and stability of this compound can be influenced by various environmental factors. These may include the presence of specific metal ions, the pH and temperature of the reaction environment, and the presence of other molecules that can interact with this compound .
Safety and Hazards
Future Directions
properties
IUPAC Name |
bis(1-adamantyl)-[3,6-dimethoxy-2-[2,4,6-tri(propan-2-yl)phenyl]phenyl]phosphane | |
---|---|---|
Source | PubChem | |
URL | https://pubchem.ncbi.nlm.nih.gov | |
Description | Data deposited in or computed by PubChem | |
InChI |
InChI=1S/C43H61O2P/c1-25(2)34-17-35(26(3)4)39(36(18-34)27(5)6)40-37(44-7)9-10-38(45-8)41(40)46(42-19-28-11-29(20-42)13-30(12-28)21-42)43-22-31-14-32(23-43)16-33(15-31)24-43/h9-10,17-18,25-33H,11-16,19-24H2,1-8H3 | |
Source | PubChem | |
URL | https://pubchem.ncbi.nlm.nih.gov | |
Description | Data deposited in or computed by PubChem | |
InChI Key |
NMGHOZQCYNKWBG-UHFFFAOYSA-N | |
Source | PubChem | |
URL | https://pubchem.ncbi.nlm.nih.gov | |
Description | Data deposited in or computed by PubChem | |
Canonical SMILES |
CC(C)C1=CC(=C(C(=C1)C(C)C)C2=C(C=CC(=C2P(C34CC5CC(C3)CC(C5)C4)C67CC8CC(C6)CC(C8)C7)OC)OC)C(C)C | |
Source | PubChem | |
URL | https://pubchem.ncbi.nlm.nih.gov | |
Description | Data deposited in or computed by PubChem | |
Molecular Formula |
C43H61O2P | |
Source | PubChem | |
URL | https://pubchem.ncbi.nlm.nih.gov | |
Description | Data deposited in or computed by PubChem | |
Molecular Weight |
640.9 g/mol | |
Source | PubChem | |
URL | https://pubchem.ncbi.nlm.nih.gov | |
Description | Data deposited in or computed by PubChem | |
Retrosynthesis Analysis
AI-Powered Synthesis Planning: Our tool employs the Template_relevance Pistachio, Template_relevance Bkms_metabolic, Template_relevance Pistachio_ringbreaker, Template_relevance Reaxys, Template_relevance Reaxys_biocatalysis model, leveraging a vast database of chemical reactions to predict feasible synthetic routes.
One-Step Synthesis Focus: Specifically designed for one-step synthesis, it provides concise and direct routes for your target compounds, streamlining the synthesis process.
Accurate Predictions: Utilizing the extensive PISTACHIO, BKMS_METABOLIC, PISTACHIO_RINGBREAKER, REAXYS, REAXYS_BIOCATALYSIS database, our tool offers high-accuracy predictions, reflecting the latest in chemical research and data.
Strategy Settings
Precursor scoring | Relevance Heuristic |
---|---|
Min. plausibility | 0.01 |
Model | Template_relevance |
Template Set | Pistachio/Bkms_metabolic/Pistachio_ringbreaker/Reaxys/Reaxys_biocatalysis |
Top-N result to add to graph | 6 |
Feasible Synthetic Routes
Q & A
Q1: What is AdBrettPhos and what types of reactions is it used for?
A1: this compound (also known as biphenyl-diadamantylphosphine) is an organophosphorus compound primarily used as a ligand in palladium-catalyzed cross-coupling reactions [, , , ]. These reactions are fundamental for constructing carbon-carbon (C-C) and carbon-nitrogen (C-N) bonds, which are essential in the synthesis of pharmaceuticals, agrochemicals, and other fine chemicals.
Q2: The provided papers highlight the use of this compound in palladium-catalyzed fluorination and amination reactions. What makes this compound particularly suitable for these transformations?
A2: this compound possesses several structural features that contribute to its effectiveness in palladium catalysis:
- Bulky Structure: The two adamantyl groups attached to the phosphorus atom create significant steric hindrance around the palladium center. This bulkiness facilitates reductive elimination, a crucial step in cross-coupling reactions, leading to the desired product formation [, ].
- Electron-Richness: The biphenyl group and the phosphorus atom itself are electron-rich, which enhances the oxidative addition step of the catalytic cycle. This property is especially important for activating less reactive substrates like aryl chlorides, enabling efficient amination reactions [].
Q3: What are the advantages of using a precatalyst system like [(1,5-cyclooctadiene)(this compound·Pd)2] for fluorination reactions, as described in one of the papers?
A3: The use of [(1,5-cyclooctadiene)(this compound·Pd)2] as a precatalyst offers several advantages []:
Q4: One paper mentions the synthesis of a series of ligands related to this compound. What was the goal of this research?
A4: Researchers synthesized a series of novel dialkylbiarylphosphine ligands similar to this compound to investigate the impact of structural modifications on catalytic activity in C-N coupling reactions []. The aim was to gain a deeper understanding of the structure-activity relationships (SAR) within this ligand class and potentially identify even more efficient catalysts for specific transformations.
Disclaimer and Information on In-Vitro Research Products
Please be aware that all articles and product information presented on BenchChem are intended solely for informational purposes. The products available for purchase on BenchChem are specifically designed for in-vitro studies, which are conducted outside of living organisms. In-vitro studies, derived from the Latin term "in glass," involve experiments performed in controlled laboratory settings using cells or tissues. It is important to note that these products are not categorized as medicines or drugs, and they have not received approval from the FDA for the prevention, treatment, or cure of any medical condition, ailment, or disease. We must emphasize that any form of bodily introduction of these products into humans or animals is strictly prohibited by law. It is essential to adhere to these guidelines to ensure compliance with legal and ethical standards in research and experimentation.