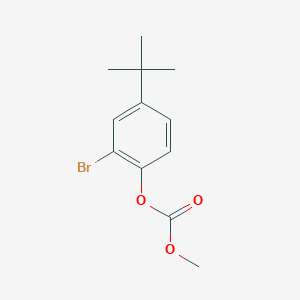
2-Bromo-4-tert-butylphenyl methyl carbonate
Overview
Description
2-Bromo-4-tert-butylphenyl methyl carbonate is an aromatic organic compound characterized by a bromine substituent at the 2-position, a bulky tert-butyl group at the 4-position, and a methyl carbonate ester functional group. This structure confers unique physicochemical properties, including moderate polarity, thermal stability, and reactivity influenced by the electron-withdrawing bromine and steric hindrance from the tert-butyl group.
Preparation Methods
Synthetic Routes and Reaction Conditions: The synthesis of 2-Bromo-4-tert-butylphenyl methyl carbonate typically involves the reaction of 2-Bromo-4-tert-butylphenol with methyl chloroformate in the presence of a base such as pyridine. The reaction is carried out under mild conditions, usually at room temperature, to ensure high yield and purity of the product.
Industrial Production Methods: Industrial production of this compound follows similar synthetic routes but on a larger scale. The process involves the use of automated reactors and precise control of reaction conditions to ensure consistent quality and yield. The use of high-purity reagents and solvents is crucial in industrial settings to minimize impurities and by-products.
Chemical Reactions Analysis
Types of Reactions: 2-Bromo-4-tert-butylphenyl methyl carbonate undergoes various chemical reactions, including:
Substitution Reactions: The bromine atom can be substituted by other nucleophiles such as amines or thiols.
Hydrolysis: The methyl carbonate group can be hydrolyzed under acidic or basic conditions to yield the corresponding phenol.
Oxidation: The compound can undergo oxidation reactions to form quinones or other oxidized derivatives.
Common Reagents and Conditions:
Substitution Reactions: Common reagents include sodium azide, potassium thiocyanate, and primary amines. These reactions are typically carried out in polar aprotic solvents such as dimethylformamide (DMF) or dimethyl sulfoxide (DMSO).
Hydrolysis: Acidic hydrolysis can be performed using hydrochloric acid, while basic hydrolysis can be achieved with sodium hydroxide.
Oxidation: Oxidizing agents such as potassium permanganate or chromium trioxide are commonly used.
Major Products Formed:
Substitution Reactions: Products include azides, thiocyanates, and substituted amines.
Hydrolysis: The major product is 2-Bromo-4-tert-butylphenol.
Oxidation: Products include quinones and other oxidized phenolic compounds.
Scientific Research Applications
2-Bromo-4-tert-butylphenyl methyl carbonate has several applications in scientific research:
Chemistry: It is used as an intermediate in the synthesis of more complex organic molecules.
Biology: The compound is used in the study of enzyme inhibition and protein-ligand interactions.
Medicine: Research is ongoing into its potential use as a pharmaceutical intermediate.
Industry: The compound is used in the production of specialty chemicals and materials.
Mechanism of Action
The mechanism by which 2-Bromo-4-tert-butylphenyl methyl carbonate exerts its effects involves its interaction with molecular targets such as enzymes and receptors. The bromine atom and tert-butyl group play crucial roles in its binding affinity and specificity. The compound can inhibit enzyme activity by forming covalent bonds with active site residues or by blocking substrate access .
Comparison with Similar Compounds
The compound’s distinct functional groups and substituents warrant comparison with three key analogs: 4-Bromo-2-tert-butylphenol, 2-Bromo-4'-methoxyacetophenone, and methyl esters.
Structural Analog: 4-Bromo-2-tert-butylphenol (CAS 10323-39-4)
This analog lacks the methyl carbonate group, instead featuring a hydroxyl (-OH) group. Key differences include:
- Reactivity: The phenolic -OH group is acidic (pKa ~10), enabling deprotonation and participation in electrophilic substitution, whereas the methyl carbonate ester undergoes hydrolysis under acidic or basic conditions to release methanol and CO₂ .
- Solubility: The phenolic -OH enhances solubility in polar solvents (e.g., water, ethanol), while the carbonate ester reduces polarity, favoring organic solvents like dichloromethane.
- Stability: The carbonate group in the target compound may confer greater stability against oxidation compared to the phenol, which is prone to quinone formation .
Brominated Aromatic Analog: 2-Bromo-4'-methoxyacetophenone (CAS 2632-13-5)
This compound shares a brominated aromatic ring but differs in substituents:
- Functional Groups: The acetophenone (C=O) and methoxy (-OCH₃) groups contrast with the tert-butyl and carbonate groups. The ketone enables nucleophilic additions (e.g., Grignard reactions), while the carbonate ester is more hydrolytically labile .
- Applications: Both compounds serve as intermediates, but the acetophenone derivative is used in synthesizing pharmaceuticals, whereas the tert-butyl group in the target compound may enhance steric control in catalysis .
Methyl Ester vs. Methyl Carbonate
While methyl esters (R-COOCH₃) and methyl carbonates (R-O-CO-OCH₃) both contain methoxy groups, their reactivity diverges:
- Hydrolysis: Methyl carbonates hydrolyze faster than esters due to the electrophilic carbonyl adjacent to two oxygen atoms, as evidenced by kinetic studies in 80% aqueous ethanol (log k = -2.1 for methyl carbonate vs. -3.5 for phenyl esters) .
- Thermal Stability : Carbonates generally exhibit lower thermal stability than esters, decomposing at ~150°C, whereas methyl esters (e.g., methyl benzoate) remain stable up to 200°C .
Table 1: Comparative Properties of 2-Bromo-4-tert-butylphenyl Methyl Carbonate and Analogs
Research Findings and Limitations
- Kinetic Behavior: The target compound’s hydrolysis rate in aqueous ethanol aligns with methyl carbonate trends, but its tert-butyl group may slow reactions due to steric effects, as inferred from log k vs. Ef plots .
- Safety Profile: Unlike 4-Bromo-2-tert-butylphenol, which requires stringent inhalation precautions, the carbonate’s lower volatility may reduce inhalation risks, though formal toxicity data are lacking .
Biological Activity
2-Bromo-4-tert-butylphenyl methyl carbonate (CAS No. 1233530-91-0) is a synthetic organic compound that has garnered attention for its potential biological activities. This article reviews the biochemical properties, cellular effects, molecular mechanisms, and relevant case studies associated with this compound.
Chemical Structure and Properties
The molecular structure of this compound features a bromine atom and a tert-butyl group attached to a phenyl ring, with a methyl carbonate functional group. This configuration influences its reactivity and biological interactions.
This compound has been studied for its role in enzyme inhibition, particularly its interaction with key metabolic enzymes. It has shown potential in inhibiting succinate dehydrogenase, an important enzyme in the citric acid cycle, which can lead to altered cellular metabolism and energy production pathways.
Cellular Effects
The compound has been observed to impact various cellular processes:
- Cell Signaling : Modulates pathways such as the mitogen-activated protein kinase (MAPK) pathway, influencing gene expression and cellular responses.
- Metabolism : Alters metabolic pathways through its inhibitory action on succinate dehydrogenase, potentially leading to therapeutic effects at specific dosages.
Molecular Mechanism
At the molecular level, this compound exerts its effects by binding to the active sites of enzymes. This binding inhibits their activity, preventing the normal catalytic processes within cells. The compound's stability under laboratory conditions suggests it can maintain its activity over time without significant degradation .
Case Studies
Several studies have explored the biological activity of this compound:
- Enzyme Inhibition Studies : Research indicates that at lower concentrations, this compound effectively inhibits succinate dehydrogenase, leading to reduced energy production in cancer cells.
- Animal Model Evaluations : In vivo studies have demonstrated that varying dosages can elicit different biological responses, with lower doses showing potential therapeutic effects while higher doses may lead to toxicity .
Comparative Analysis of Biological Activity
The following table summarizes some key findings related to the biological activity of this compound compared to other compounds:
Compound | IC50 (μM) | Biological Activity | Notes |
---|---|---|---|
This compound | TBD | Enzyme inhibition | Affects succinate dehydrogenase |
Compound A (e.g., Novel Inhibitor) | 0.34 | Potent CYP3A4 inhibitor | High risk for drug-drug interactions |
Compound B (e.g., Anticancer Agent) | 19 | Selective cytotoxicity | Effective against T-lymphoblastic lines |
Q & A
Q. What are the established synthetic routes for 2-Bromo-4-tert-butylphenyl methyl carbonate?
Basic
The synthesis typically involves two key steps: (1) bromination of 4-tert-butylphenol derivatives and (2) carbonate formation. For bromination, electrophilic substitution using N-bromosuccinimide (NBS) under acidic conditions is common. The carbonate group is introduced via reaction with methyl chloroformate in the presence of a base (e.g., pyridine) to neutralize HCl byproducts. Purification often employs column chromatography with gradients of ethyl acetate/hexane .
Q. How can computational methods like DFT predict the reactivity of this compound in nucleophilic substitution reactions?
Advanced
Density Functional Theory (DFT) calculations, such as those based on the Colle-Salvetti correlation-energy functional, can model electron density distribution and local kinetic energy to predict sites of nucleophilic attack. For instance, the bromine atom’s electrophilicity and the carbonate group’s steric hindrance (due to the tert-butyl group) can be quantified. Transition state analysis reveals activation barriers for SN2 mechanisms, while Fukui indices identify regions susceptible to nucleophilic substitution .
Q. What spectroscopic techniques are most effective for characterizing this compound?
Basic
- NMR : and NMR identify substituents (e.g., tert-butyl at δ ~1.3 ppm, methyl carbonate at δ ~3.8 ppm).
- FTIR : C=O stretching (~1750 cm) confirms the carbonate group; C-Br vibration appears near 600 cm.
- Mass Spectrometry : High-resolution MS (HRMS) verifies molecular ion peaks (e.g., [M+Na]) and fragmentation patterns .
Q. How to resolve discrepancies in NMR data when analyzing this compound synthesized via different methods?
Advanced
Discrepancies may arise from solvent polarity, diastereomer formation, or residual catalysts. For example, polar aprotic solvents (e.g., DMSO) can shift proton signals. Use deuterated solvents and compare with computational NMR predictions (e.g., GIAO method). Impurities from incomplete purification can be addressed via iterative recrystallization or preparative HPLC. Cross-validation with X-ray crystallography (e.g., SHELX refinement) resolves structural ambiguities .
Q. What factors influence the thermal stability of this compound under varying conditions?
Advanced
Thermogravimetric analysis (TGA) reveals decomposition onset temperatures. The tert-butyl group enhances steric protection, delaying thermal breakdown. However, the carbonate ester is prone to hydrolysis in humid environments. Kinetic studies under controlled humidity (e.g., 40–80% RH) quantify degradation rates. Accelerated stability testing (40–60°C) models long-term storage conditions, with HPLC monitoring degradation products like 4-tert-butylphenol .
Q. What role does the tert-butyl group play in the steric and electronic effects during Suzuki-Miyaura coupling reactions?
Advanced
The tert-butyl group introduces steric hindrance, slowing oxidative addition of the palladium catalyst to the C-Br bond. Electronically, it slightly deactivates the aryl ring via inductive effects, reducing coupling efficiency. Computational studies (e.g., molecular volume calculations) optimize ligand choice (e.g., SPhos) to mitigate steric effects. Reaction optimization may require elevated temperatures (80–100°C) and prolonged reaction times .
Q. How to optimize reaction conditions for synthesizing this compound to minimize by-products?
Advanced
- Catalyst Screening : Use DMAP (4-dimethylaminopyridine) to accelerate carbonate formation while reducing side reactions.
- Solvent Selection : Anhydrous dichloromethane minimizes hydrolysis.
- Temperature Control : Maintain 0–5°C during methyl chloroformate addition to suppress esterification by-products.
- In Situ Monitoring : ReactIR tracks carbonate formation in real time, enabling rapid adjustment of reagent stoichiometry .
Properties
IUPAC Name |
(2-bromo-4-tert-butylphenyl) methyl carbonate | |
---|---|---|
Source | PubChem | |
URL | https://pubchem.ncbi.nlm.nih.gov | |
Description | Data deposited in or computed by PubChem | |
InChI |
InChI=1S/C12H15BrO3/c1-12(2,3)8-5-6-10(9(13)7-8)16-11(14)15-4/h5-7H,1-4H3 | |
Source | PubChem | |
URL | https://pubchem.ncbi.nlm.nih.gov | |
Description | Data deposited in or computed by PubChem | |
InChI Key |
CTCBHKRFIIJJOA-UHFFFAOYSA-N | |
Source | PubChem | |
URL | https://pubchem.ncbi.nlm.nih.gov | |
Description | Data deposited in or computed by PubChem | |
Canonical SMILES |
CC(C)(C)C1=CC(=C(C=C1)OC(=O)OC)Br | |
Source | PubChem | |
URL | https://pubchem.ncbi.nlm.nih.gov | |
Description | Data deposited in or computed by PubChem | |
Molecular Formula |
C12H15BrO3 | |
Source | PubChem | |
URL | https://pubchem.ncbi.nlm.nih.gov | |
Description | Data deposited in or computed by PubChem | |
Molecular Weight |
287.15 g/mol | |
Source | PubChem | |
URL | https://pubchem.ncbi.nlm.nih.gov | |
Description | Data deposited in or computed by PubChem | |
Synthesis routes and methods
Procedure details
Retrosynthesis Analysis
AI-Powered Synthesis Planning: Our tool employs the Template_relevance Pistachio, Template_relevance Bkms_metabolic, Template_relevance Pistachio_ringbreaker, Template_relevance Reaxys, Template_relevance Reaxys_biocatalysis model, leveraging a vast database of chemical reactions to predict feasible synthetic routes.
One-Step Synthesis Focus: Specifically designed for one-step synthesis, it provides concise and direct routes for your target compounds, streamlining the synthesis process.
Accurate Predictions: Utilizing the extensive PISTACHIO, BKMS_METABOLIC, PISTACHIO_RINGBREAKER, REAXYS, REAXYS_BIOCATALYSIS database, our tool offers high-accuracy predictions, reflecting the latest in chemical research and data.
Strategy Settings
Precursor scoring | Relevance Heuristic |
---|---|
Min. plausibility | 0.01 |
Model | Template_relevance |
Template Set | Pistachio/Bkms_metabolic/Pistachio_ringbreaker/Reaxys/Reaxys_biocatalysis |
Top-N result to add to graph | 6 |
Feasible Synthetic Routes
Disclaimer and Information on In-Vitro Research Products
Please be aware that all articles and product information presented on BenchChem are intended solely for informational purposes. The products available for purchase on BenchChem are specifically designed for in-vitro studies, which are conducted outside of living organisms. In-vitro studies, derived from the Latin term "in glass," involve experiments performed in controlled laboratory settings using cells or tissues. It is important to note that these products are not categorized as medicines or drugs, and they have not received approval from the FDA for the prevention, treatment, or cure of any medical condition, ailment, or disease. We must emphasize that any form of bodily introduction of these products into humans or animals is strictly prohibited by law. It is essential to adhere to these guidelines to ensure compliance with legal and ethical standards in research and experimentation.