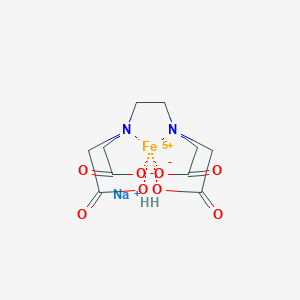
Sodium feredetate
Overview
Description
Sodium feredetate (C₁₀H₁₂FeN₂NaO₈; molecular weight 367.047 g/mol) is a trivalent iron chelate complexed with ethylenediaminetetraacetic acid (EDTA). It is classified under the Anatomical Therapeutic Chemical (ATC) code B03AB03 as an oral iron preparation for treating iron-deficiency anemia (IDA) . Unlike traditional iron salts, this compound’s EDTA chelation prevents iron ionization, enhancing stability and bioavailability while reducing gastrointestinal (GI) irritation and tooth discoloration . It is available as a liquid formulation (e.g., Sytron®) and tablets, often combined with vitamin B₁₂ and folate .
Preparation Methods
Synthetic Routes for Sodium Feredetate Preparation
Chelation of Ferric Chloride with EDTA Tetrasodium
The most widely documented method involves the reaction of ferric chloride (FeCl₃) with EDTA tetrasodium in aqueous media. This approach capitalizes on the high affinity of EDTA for ferric ions, forming a stable octahedral complex. The stoichiometric ratio of EDTA tetrasodium to FeCl₃ is critical, with optimal molar ratios ranging from 1:1 to 1.15:1 to ensure complete chelation and minimize free iron residues .
Reaction Equation :
4\text{EDTA} + \text{FeCl}3 \rightarrow \text{NaFe(EDTA)} + 3\text{NaCl}
The process begins by dissolving EDTA tetrasodium in deionized water at 60–80°C, followed by gradual addition of FeCl₃ under vigorous stirring. Maintaining a pH of 4–7 prevents hydrolysis of ferric ions, which could lead to insoluble ferric hydroxides . Post-reaction, the solution is filtered hot to remove particulate matter, and crystallization is induced by cooling to 20–50°C with seed crystals.
Key Parameters:
Direct Synthesis from Elemental Iron and EDTA
An alternative method employs elemental iron (reduced or electrolytic grade) as the iron source, which reacts with hydrochloric acid (HCl) to generate ferrous ions in situ. Subsequent oxidation to ferric ions and chelation with EDTA occurs under controlled conditions .
Reaction Steps :
-
Iron Dissolution :
2 + \text{H}2\uparrow
-
Oxidation and Chelation :
2 + \text{O}2 + 4\text{HCl} \rightarrow 4\text{FeCl}3 + 2\text{H}2\text{O}
3 + \text{Na}4\text{EDTA} \rightarrow \text{NaFe(EDTA)} + 3\text{NaCl}
This method avoids the use of pre-formed ferric salts, reducing costs. However, precise control of oxidation conditions (e.g., aeration rate, temperature) is necessary to prevent over-oxidation or incomplete chelation.
Key Parameters:
-
HCl Concentration : 10–15% (w/v)
-
Reaction Temperature : 35–60°C
Reaction Parameter Optimization
Temperature and pH Dependence
The solubility of EDTA and iron species is highly temperature-dependent. Elevated temperatures (≥60°C) enhance reaction rates but risk EDTA decomposition above 100°C. A pH range of 4–7 balances chelation efficiency and stability, as acidic conditions favor Fe³⁺ solubility, while neutral pH minimizes side reactions .
Crystallization and Purification
Post-synthesis, this compound is isolated via cooling crystallization . Seed crystals (0.5–2% w/w) are introduced at 40–50°C to nucleate homogeneous crystal growth. The product is then vacuum-dried at 50–60°C to remove residual moisture without decomposing the complex .
Industrial Production Considerations
Batch vs. Continuous Processes
Industrial-scale production typically employs batch reactors (500–10,000 L capacity) due to easier control over reaction parameters. However, continuous flow systems are being explored to improve yield consistency and reduce energy consumption.
Table 1: Comparison of Synthesis Methods
Parameter | FeCl₃ Method | Elemental Iron Method |
---|---|---|
Raw Material Cost | High (FeCl₃) | Low (Fe⁰) |
Reaction Time | 1–3 hours | 2–4 hours |
Byproducts | NaCl | NaCl, H₂ |
Scalability | High | Moderate |
Purity | ≥98% | ≥95% |
Case Studies from Patent Literature
Example 1: FeCl₃-Based Synthesis
-
EDTA Tetrasodium : 45.2 g (0.1 mol)
-
FeCl₃ : 27 g (0.1 mol)
-
Water : 120 mL
-
Conditions : 65°C, 1.5 hours, pH 5.2
-
Yield : 44.7 g (89%)
Example 2: Elemental Iron Synthesis
-
Iron Powder : 30 g (0.54 mol)
-
HCl (15%) : 250 mL
-
EDTA : 75 g (0.2 mol)
-
Conditions : 50°C, 1 hour, pH 4.5
-
Yield : 106 g (85%)
Challenges and Mitigation Strategies
Impurity Control
-
Unreacted EDTA : Minimized by maintaining stoichiometric excess of Fe³⁺.
-
Ferric Hydroxides : Prevented through pH control and rapid mixing.
Environmental Impact
The FeCl₃ method generates NaCl wastewater, requiring desalination before discharge. The elemental iron route produces H₂ gas, necessitating explosion-proof equipment.
Chemical Reactions Analysis
Types of Reactions
Sodium feredetate undergoes various chemical reactions, including:
Oxidation-Reduction Reactions: It can participate in redox reactions where the ferric ion (Fe^3+) is reduced to ferrous ion (Fe^2+).
Substitution Reactions: The EDTA ligand can be replaced by other ligands in the presence of stronger chelating agents.
Common Reagents and Conditions
Oxidation-Reduction: Common reagents include reducing agents like ascorbic acid and oxidizing agents like hydrogen peroxide.
Substitution: Strong chelating agents such as ethylenediamine or diethylenetriamine can be used under controlled pH conditions.
Major Products
Redox Reactions: The major products include ferrous EDTA and free radicals.
Substitution Reactions: The products depend on the substituting ligand but generally include new metal-ligand complexes.
Scientific Research Applications
Agricultural Applications
Sodium feredetate is primarily used in agriculture to address iron deficiency in plants, particularly in soils with high pH levels where iron becomes insoluble.
- Iron Chelation : By chelating iron, this compound increases the bioavailability of iron to plants, effectively preventing iron chlorosis—a condition where leaves turn yellow due to insufficient iron.
- Micronutrient Fertilizer : It is often included in micronutrient fertilizers to improve crop yield and quality, especially in crops like fruits and vegetables that are sensitive to iron deficiency.
Medical Applications
In medicine, this compound has been studied for its role in treating iron deficiency anemia.
- Iron Supplementation : Clinical studies have demonstrated that this compound is effective in increasing hemoglobin levels in patients with iron deficiency anemia. For instance, a study showed that 82.5% of patients treated with Fedate syrup (this compound) experienced significant increases in hemoglobin levels within 21 days .
- Comparative Efficacy : Research comparing this compound to traditional iron supplements like ferrous sulfate indicates that it may produce quicker and more significant increases in hemoglobin levels with fewer side effects .
Environmental Applications
This compound is also utilized in environmental science for its ability to sequester heavy metals.
- Water Treatment : It can be employed in water treatment processes to remove heavy metals from contaminated water sources. The chelation properties help solubilize heavy metals, making them easier to filter out.
- Analytical Chemistry : In laboratory settings, this compound is used as a reagent for the analysis of metal ions in various samples, aiding in environmental monitoring and research.
Case Study 1: Efficacy in Pregnant Women
A clinical trial involving pregnant women with iron deficiency anemia found that this compound significantly improved hemoglobin levels compared to ferrous sulfate. The study reported an average increase of 2.11 g/dL after two months of treatment with this compound, highlighting its effectiveness and better tolerability .
Case Study 2: Agricultural Impact
In agricultural trials, the application of this compound as a foliar spray resulted in improved leaf chlorophyll content and overall plant health in crops affected by iron chlorosis. This led to increased fruit yield and quality, demonstrating its practical benefits for farmers.
Data Table: Comparison of this compound and Ferrous Sulfate
Parameter | This compound | Ferrous Sulfate |
---|---|---|
Average Hb Increase (g/dL) | 2.11 after 2 months | 1.9 after 2 months |
Side Effects | Minimal | Moderate |
Bioavailability | High | Moderate |
Application | Oral | Oral |
Mechanism of Action
Sodium feredetate works by binding to metal ions through its multiple carboxylate and amine groups, forming stable, water-soluble complexes. This chelation process prevents metal ions from precipitating and makes them bioavailable for various biological and chemical processes. In agriculture, it helps plants absorb iron more efficiently, while in medicine, it enhances the bioavailability of iron in fortified foods.
Comparison with Similar Compounds
Comparative Efficacy with Traditional Iron Salts
Table 1: Efficacy of Sodium Feredetate vs. Ferrous Salts in IDA
Key Observations :
- This compound achieves comparable or superior hemoglobin (Hb) restoration at lower elemental iron doses than ferrous salts .
- In a UK study, this compound (33 mg/day) increased Hb by 2.11 g/dL over two months, outperforming ferrous sulfate (1.58 g/dL) despite half the iron dose .
Comparison with Novel Iron Formulations
Table 2: this compound vs. Novel Iron Agents
Key Observations :
- Ferric maltol: Licensed for IBD-associated anemia, it shows moderate bioavailability but requires longer treatment (12+ weeks) for sustained Hb normalization. It is less effective than intravenous iron .
- Sucrosomial iron : Its unique "sucrosome" structure enables high absorption with minimal GI side effects. At 30–60 mg/day, it outperforms ferrous sulfate (105–210 mg/day) in Hb rise (2.7 vs. 1.4 g/dL) .
- This compound’s EDTA chelation provides a balance of high bioavailability and tolerability, though newer agents like sucrosomial iron may offer enhanced absorption .
Pharmacokinetic and Pharmacodynamic Considerations
- Absorption : The EDTA-iron chelate dissociates in the GI tract, releasing elemental iron for absorption. Absorption is enhanced in iron-deficient states .
- Distribution : Post-absorption, 60–70% of iron integrates into hemoglobin, with the remainder stored as ferritin or haemosiderin .
- Manufacturing : High-purity synthesis (99.9% purity) ensures minimal heavy metal residues, making it suitable for pharmaceutical use .
Q & A
Basic Research Questions
Q. What are the validated methods for synthesizing sodium feredetate (C₁₀H₁₂FeN₂NaO₈) with high purity, and how can impurities be minimized during synthesis?
- Methodological Answer : Synthesis typically involves chelation of ferric ions with ethylenediaminetetraacetic acid (EDTA) derivatives under controlled pH and temperature. Purification steps, such as recrystallization from aqueous ethanol, are critical to minimize residual solvents or unreacted precursors. Characterization via X-ray diffraction (XRD) and nuclear magnetic resonance (NMR) ensures structural fidelity. For reproducibility, document reaction stoichiometry, solvent ratios, and crystallization conditions in detail .
- Data Reliability : Small-scale synthesis (common in academic settings) may limit analytical data; use corrected purity values (e.g., HPLC with adjustments for residual solvents) rather than raw chromatographic results .
Q. How should this compound’s stability be assessed under varying storage conditions (e.g., temperature, humidity)?
- Methodological Answer : Conduct accelerated stability studies using International Council for Harmonisation (ICH) guidelines. Monitor degradation via UV-Vis spectroscopy for Fe³⁺ release or HPLC for organic byproducts. Include control samples stored at -20°C as a baseline. For short-term studies, use thermal gravimetric analysis (TGA) to assess hygroscopicity .
Q. What spectroscopic and chromatographic techniques are most effective for characterizing this compound’s structure and purity?
- Methodological Answer : Pair Fourier-transform infrared spectroscopy (FTIR) with EDTA-specific ligand vibration bands (~1600 cm⁻¹) to confirm chelation. High-resolution mass spectrometry (HRMS) validates molecular weight (367.05 g/mol). For purity, combine reverse-phase HPLC with inductively coupled plasma mass spectrometry (ICP-MS) to quantify Fe³⁺ content .
Advanced Research Questions
Q. How can computational models (e.g., DFT, molecular dynamics) predict this compound’s reactivity in biological systems?
- Methodological Answer : Density functional theory (DFT) optimizes the molecule’s geometry to identify reactive sites (e.g., Fe³⁺ coordination). Molecular dynamics (MD) simulations in explicit solvent models (e.g., SPC/E water) can predict ligand-exchange kinetics. Validate predictions with in vitro assays measuring iron release rates .
Q. What experimental designs resolve contradictions in reported pharmacological efficacy (e.g., iron bioavailability vs. toxicity)?
- Methodological Answer : Use a factorial design to isolate variables (e.g., dosage, administration route, cellular uptake mechanisms). Compare in vivo models (e.g., murine iron-deficiency anemia) with in vitro Caco-2 cell assays to differentiate systemic vs. cellular effects. Employ meta-analysis to reconcile disparate datasets .
Q. How do pH and redox conditions influence this compound’s iron release mechanism, and how can this be quantified experimentally?
- Methodological Answer : Design pH-dependent kinetic studies using stopped-flow spectrophotometry to monitor Fe³⁺ dissociation rates. Electrochemical methods (cyclic voltammetry) assess redox stability. Data interpretation should account for competing ligands (e.g., plasma proteins) using conditional stability constants (log K) .
Q. Data Analysis & Reproducibility
Q. What statistical approaches are recommended for analyzing dose-response relationships in this compound studies?
- Methodological Answer : Apply nonlinear regression (e.g., Hill equation) to model dose-response curves. Use ANOVA with post-hoc Tukey tests for multi-group comparisons. For small sample sizes, bootstrap resampling reduces type I/II errors. Report effect sizes (e.g., Cohen’s d) to contextualize biological significance .
Q. How can researchers ensure reproducibility when scaling up this compound synthesis from milligram to gram quantities?
- Methodological Answer : Maintain strict control over mixing rates and temperature gradients during scaling. Use process analytical technology (PAT) tools like in-situ Raman spectroscopy to monitor reaction progress. Publish detailed protocols, including raw material sources and equipment specifications (e.g., reactor geometry) .
Q. Ethical & Reporting Standards
Q. What guidelines should be followed when reporting this compound’s toxicological data in peer-reviewed journals?
- Methodological Answer : Adhere to OECD Test Guidelines (e.g., TG 423 for acute oral toxicity) and ARRIVE 2.0 guidelines for in vivo studies. Disclose batch-specific purity data and storage conditions. Use FAIR data principles (Findable, Accessible, Interoperable, Reusable) for supplementary datasets .
Properties
CAS No. |
15708-41-5 |
---|---|
Molecular Formula |
C10H12FeN2NaO8 |
Molecular Weight |
367.05 g/mol |
IUPAC Name |
sodium;2-[2-[bis(carboxylatomethyl)amino]ethyl-(carboxylatomethyl)amino]acetate;iron(3+) |
InChI |
InChI=1S/C10H16N2O8.Fe.Na/c13-7(14)3-11(4-8(15)16)1-2-12(5-9(17)18)6-10(19)20;;/h1-6H2,(H,13,14)(H,15,16)(H,17,18)(H,19,20);;/q;+3;+1/p-4 |
InChI Key |
MKWYFZFMAMBPQK-UHFFFAOYSA-J |
SMILES |
C(CN(CC(=O)[O-])CC(=O)[O-])N(CC(=O)[O-])CC(=O)[O-].[Na+].[Fe+3] |
Canonical SMILES |
C(CN(CC(=O)[O-])CC(=O)[O-])N(CC(=O)[O-])CC(=O)[O-].[Na+].[Fe+3] |
Key on ui other cas no. |
15708-41-5 |
physical_description |
Light yellow solid; [JECFA] Yellowish-brown odorless powder; Insoluble in water; |
Pictograms |
Irritant |
Related CAS |
15275-07-7 (Parent) |
Synonyms |
ammonium ferric edetate EDTA Fe(III) EDTA ferric ammonium Fe(III)-EDTA Fe(III)-edta complex (1:1) Fe(III)-EDTA, ammonium salt Fe(III)-EDTA, potassium salt Fe(III)-EDTA, sodium salt Fe(III)-EDTA, sodium salt, trihydrate ferrate(1-), ((ethylenedinitrilo)tetraacetato)-, hydrogen ferric EDTA ferric sodium edetate ferric-edta hydrogen ((ethylenedinitrilo)tetraacetato)ferrate(III) iron(III) EDTA Irostrene monoferric edetate NaFeEDTA sodium feredetate sodium iron EDTA Sytron |
Origin of Product |
United States |
Retrosynthesis Analysis
AI-Powered Synthesis Planning: Our tool employs the Template_relevance Pistachio, Template_relevance Bkms_metabolic, Template_relevance Pistachio_ringbreaker, Template_relevance Reaxys, Template_relevance Reaxys_biocatalysis model, leveraging a vast database of chemical reactions to predict feasible synthetic routes.
One-Step Synthesis Focus: Specifically designed for one-step synthesis, it provides concise and direct routes for your target compounds, streamlining the synthesis process.
Accurate Predictions: Utilizing the extensive PISTACHIO, BKMS_METABOLIC, PISTACHIO_RINGBREAKER, REAXYS, REAXYS_BIOCATALYSIS database, our tool offers high-accuracy predictions, reflecting the latest in chemical research and data.
Strategy Settings
Precursor scoring | Relevance Heuristic |
---|---|
Min. plausibility | 0.01 |
Model | Template_relevance |
Template Set | Pistachio/Bkms_metabolic/Pistachio_ringbreaker/Reaxys/Reaxys_biocatalysis |
Top-N result to add to graph | 6 |
Feasible Synthetic Routes
Disclaimer and Information on In-Vitro Research Products
Please be aware that all articles and product information presented on BenchChem are intended solely for informational purposes. The products available for purchase on BenchChem are specifically designed for in-vitro studies, which are conducted outside of living organisms. In-vitro studies, derived from the Latin term "in glass," involve experiments performed in controlled laboratory settings using cells or tissues. It is important to note that these products are not categorized as medicines or drugs, and they have not received approval from the FDA for the prevention, treatment, or cure of any medical condition, ailment, or disease. We must emphasize that any form of bodily introduction of these products into humans or animals is strictly prohibited by law. It is essential to adhere to these guidelines to ensure compliance with legal and ethical standards in research and experimentation.