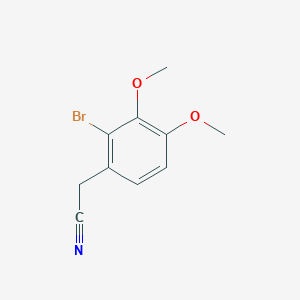
2-(2-Bromo-3,4-dimethoxyphenyl)acetonitrile
- Click on QUICK INQUIRY to receive a quote from our team of experts.
- With the quality product at a COMPETITIVE price, you can focus more on your research.
Overview
Description
2-(2-Bromo-3,4-dimethoxyphenyl)acetonitrile is an organic compound with the molecular formula C10H10BrNO2 It is a derivative of acetonitrile, where the phenyl ring is substituted with bromine and methoxy groups
Preparation Methods
Synthetic Routes and Reaction Conditions
The synthesis of 2-(2-Bromo-3,4-dimethoxyphenyl)acetonitrile typically involves the bromination of 3,4-dimethoxyphenylacetonitrile. The reaction is carried out under controlled conditions to ensure selective bromination at the desired position. Common reagents used in this process include bromine or N-bromosuccinimide (NBS) in the presence of a suitable solvent such as dichloromethane or acetonitrile .
Industrial Production Methods
Industrial production methods for this compound are similar to laboratory synthesis but are scaled up to accommodate larger quantities. The process involves the use of industrial-grade reagents and solvents, and the reaction conditions are optimized for efficiency and yield. The product is typically purified through recrystallization or distillation .
Chemical Reactions Analysis
Types of Reactions
2-(2-Bromo-3,4-dimethoxyphenyl)acetonitrile undergoes various chemical reactions, including:
Substitution Reactions: The bromine atom can be replaced by other nucleophiles such as amines or thiols.
Oxidation Reactions: The compound can be oxidized to form corresponding aldehydes or carboxylic acids.
Reduction Reactions: The nitrile group can be reduced to form amines.
Common Reagents and Conditions
Substitution: Reagents like sodium azide or potassium thiocyanate in polar solvents.
Oxidation: Reagents such as potassium permanganate or chromium trioxide.
Reduction: Catalysts like palladium on carbon (Pd/C) or lithium aluminum hydride (LiAlH4).
Major Products
Substitution: Formation of substituted phenylacetonitriles.
Oxidation: Formation of 2-(2-bromo-3,4-dimethoxyphenyl)acetaldehyde or 2-(2-bromo-3,4-dimethoxyphenyl)acetic acid.
Reduction: Formation of 2-(2-bromo-3,4-dimethoxyphenyl)ethylamine.
Scientific Research Applications
2-(2-Bromo-3,4-dimethoxyphenyl)acetonitrile has several applications in scientific research:
Chemistry: Used as an intermediate in the synthesis of complex organic molecules.
Biology: Studied for its potential biological activities and interactions with biomolecules.
Medicine: Investigated for its potential use in drug development and as a precursor for pharmaceutical compounds.
Industry: Utilized in the production of specialty chemicals and materials.
Mechanism of Action
The mechanism of action of 2-(2-Bromo-3,4-dimethoxyphenyl)acetonitrile involves its interaction with molecular targets such as enzymes and receptors. The bromine and methoxy groups play a crucial role in modulating the compound’s reactivity and binding affinity. The nitrile group can participate in nucleophilic addition reactions, leading to the formation of various bioactive compounds .
Comparison with Similar Compounds
Similar Compounds
3,4-Dimethoxyphenylacetonitrile: Lacks the bromine substitution, leading to different reactivity and applications.
2-Bromo-4,5-dimethoxyphenylacetonitrile: Similar structure but with different substitution pattern, affecting its chemical properties and uses.
Properties
CAS No. |
72912-39-1 |
---|---|
Molecular Formula |
C10H10BrNO2 |
Molecular Weight |
256.10 g/mol |
IUPAC Name |
2-(2-bromo-3,4-dimethoxyphenyl)acetonitrile |
InChI |
InChI=1S/C10H10BrNO2/c1-13-8-4-3-7(5-6-12)9(11)10(8)14-2/h3-4H,5H2,1-2H3 |
InChI Key |
DUDRWPAFARHZKZ-UHFFFAOYSA-N |
Canonical SMILES |
COC1=C(C(=C(C=C1)CC#N)Br)OC |
Origin of Product |
United States |
Disclaimer and Information on In-Vitro Research Products
Please be aware that all articles and product information presented on BenchChem are intended solely for informational purposes. The products available for purchase on BenchChem are specifically designed for in-vitro studies, which are conducted outside of living organisms. In-vitro studies, derived from the Latin term "in glass," involve experiments performed in controlled laboratory settings using cells or tissues. It is important to note that these products are not categorized as medicines or drugs, and they have not received approval from the FDA for the prevention, treatment, or cure of any medical condition, ailment, or disease. We must emphasize that any form of bodily introduction of these products into humans or animals is strictly prohibited by law. It is essential to adhere to these guidelines to ensure compliance with legal and ethical standards in research and experimentation.