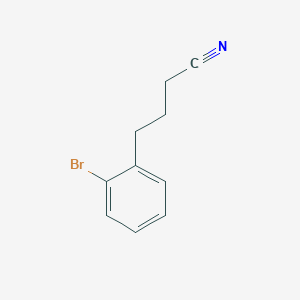
4-(2-Bromophenyl)butanenitrile
Overview
Description
4-(2-Bromophenyl)butanenitrile (CAS: 178809-32-0) is a nitrile derivative with a brominated aromatic ring attached to a four-carbon aliphatic chain. Its molecular formula is C₁₀H₁₀BrN, and its structure features a bromine atom at the ortho position of the phenyl group and a nitrile (-CN) group at the terminal end of the butane chain . This compound is primarily used in synthetic organic chemistry as an intermediate for pharmaceuticals, agrochemicals, and materials science due to its versatile reactivity, particularly in cross-coupling reactions and nucleophilic substitutions .
Key properties include:
Preparation Methods
Synthetic Routes and Reaction Conditions
Direct Bromination of Phenylbutanenitrile Derivatives
One approach involves the bromination of phenylbutanenitrile derivatives under controlled conditions to selectively introduce bromine at the ortho position. This method requires careful control of reaction parameters to avoid polybromination or substitution at undesired positions.
- Reagents: Bromine or bromide sources (e.g., N-bromosuccinimide, molecular bromine)
- Catalysts: Lewis acids or radical initiators may be employed to enhance selectivity
- Solvents: Polar aprotic solvents such as dichloromethane or acetonitrile
- Temperature: Typically maintained at low to moderate temperatures (0–40 °C) to control regioselectivity
Note: This method can suffer from regioselectivity challenges and requires purification steps to isolate the ortho-bromo isomer.
Nucleophilic Substitution Using 2-Bromophenyl Precursors
A more common and efficient method involves the nucleophilic substitution reaction between a 2-bromophenyl derivative and a suitable butanenitrile-containing alkyl halide under basic conditions.
- Typical Reaction: 2-bromophenyl nucleophile reacts with 4-bromobutanenitrile or equivalent alkyl halide
- Base: Potassium carbonate or sodium hydride to deprotonate and activate nucleophile
- Solvent: Polar aprotic solvents such as dimethylformamide (DMF) or dimethyl sulfoxide (DMSO)
- Temperature: Elevated temperatures (80–120 °C) to facilitate substitution
- Reaction Time: Several hours (4–24 h) depending on scale and conditions
This approach is favored for its higher yields, better regioselectivity, and scalability.
Comparative Table of Preparation Methods
Methodology | Key Reagents & Catalysts | Solvent | Temperature Range (°C) | Yield (%) | Notes |
---|---|---|---|---|---|
Direct Bromination | Bromine, Lewis acid catalysts | DCM, Acetonitrile | 0–40 | Variable | Regioselectivity challenges |
Nucleophilic Substitution | 2-Bromophenyl derivative, K2CO3 | DMF, DMSO | 80–120 | 75–90 | High regioselectivity, scalable |
Phase Transfer Catalysis Enhancement | Benzalkonium bromide, NaOH | Biphasic aqueous/organic | ≤90 | Increased | Improved reaction rate and yield |
Example Preparation Procedure (Adapted from Patent Literature)
Step 1: In a reaction vessel, 2-bromophenyl derivative is dissolved in dimethylformamide.
Step 2: Potassium carbonate is added as a base, followed by the slow addition of 4-bromobutanenitrile under stirring.
Step 3: The reaction mixture is heated to 90 °C and stirred for 4–6 hours.
Step 4: After completion, the reaction mixture is cooled, and water is added to precipitate the product.
Step 5: The precipitate is filtered, washed with water, and dried to yield 4-(2-bromophenyl)butanenitrile as a neutral crystalline solid.
Notes on Related Bromophenyl Derivative Syntheses
- While direct literature on this compound is limited, analogous compounds such as 4-bromo-2,2-diphenylbutyronitrile have been prepared using similar catalytic and alkaline conditions, providing a useful reference for reaction optimization.
- The control of bromination steps is critical as the formation of dibromo derivatives (e.g., 2,4-dibromo compounds) can complicate purification and reduce yield.
Scientific Research Applications
Organic Synthesis
4-(2-Bromophenyl)butanenitrile serves as a versatile building block in organic synthesis. It is often utilized in the synthesis of various organic compounds, including pharmaceuticals and agrochemicals.
Case Study: Synthesis of Novel Compounds
In a study published in the Journal of Organic Chemistry, researchers utilized this compound to synthesize new derivatives with potential biological activity. The compound was reacted with various nucleophiles, leading to the formation of substituted products that exhibited enhanced pharmacological properties .
Materials Science
The compound is also explored for its applications in materials science, particularly in the development of organic light-emitting diodes (OLEDs) and organic photovoltaics (OPVs).
Case Study: OLED Applications
Research indicates that derivatives of this compound can be integrated into OLED devices due to their favorable electronic properties. For instance, a study demonstrated that incorporating this compound into a polymer matrix improved the device's efficiency and stability .
Property | Value |
---|---|
Emission Color | Red |
Maximum Brightness | 10,034 cd/m² |
Efficiency | 2.4% |
Medicinal Chemistry
In medicinal chemistry, this compound is being investigated for its potential therapeutic effects.
Case Study: Anticancer Activity
A recent study highlighted the compound's role as an intermediate in synthesizing compounds with anticancer properties. By modifying the nitrile group, researchers were able to enhance the cytotoxic effects against specific cancer cell lines .
Coordination Chemistry
The compound has been used as a ligand in coordination chemistry, particularly in forming metal complexes which exhibit interesting catalytic properties.
Case Study: Catalytic Applications
In coordination with transition metals, this compound has shown promise as a catalyst for various reactions, including cross-coupling reactions which are crucial in synthesizing complex organic molecules .
Mechanism of Action
The mechanism of action of 4-(2-Bromophenyl)butanenitrile depends on its specific application. In medicinal chemistry, it may interact with biological targets such as enzymes or receptors, leading to modulation of their activity. The bromine atom and nitrile group play crucial roles in these interactions, influencing binding affinity and specificity.
Comparison with Similar Compounds
Para-Substituted Bromophenylbutanenitriles
- 4-(4-Bromophenyl)butanenitrile (4c) :
- Structure : Bromine at the para position of the phenyl group.
- Molecular formula : C₁₀H₁₀BrN (same as 4-(2-bromophenyl)butanenitrile).
- Key differences :
- The para-bromo substitution reduces steric hindrance compared to the ortho isomer, facilitating reactions like Suzuki-Miyaura couplings.
- Higher melting point (80.5–82.2°C) due to symmetrical crystal packing .
Di-Substituted Aromatic Derivatives
- 4-(4-Bromo-2-fluorophenyl)butanenitrile :
- Structure : Bromine at para and fluorine at ortho positions.
- Molecular formula : C₁₀H₉BrFN.
- Key differences :
- Fluorine's electron-withdrawing effect enhances the nitrile's electrophilicity, making it more reactive in nucleophilic additions.
- Higher molecular weight (332.02 g/mol) and density compared to mono-substituted analogs . Applications: Potential use in fluorinated drug candidates .
Functionalized Butanenitriles with Heteroatoms
- 4-((2-((4-Bromophenyl)thio)ethyl)dimethylsilyl)butanenitrile (11) :
- Structure : Contains a thioether (-S-) and dimethylsilyl (-Si(CH₃)₂-) group.
- Molecular formula : C₁₅H₂₁BrNSSi.
- Key differences :
- The sulfur atom introduces susceptibility to oxidation, forming sulfoxides or sulfones.
The silyl group improves thermal stability, making it suitable for high-temperature reactions .
- 4-[4-(1-Cyanodimethylamino)phenyl]butanenitrile (95): Structure: Features a dimethylamino (-N(CH₃)₂) and cyano (-CN) group on the phenyl ring. Molecular formula: C₁₃H₁₄N₄. Key differences:
- The electron-donating dimethylamino group increases the aromatic ring's electron density, altering reactivity in electrophilic substitutions.
- Used as a precursor for histamine receptor ligands .
Structural Isomers and Short-Chain Analogs
- 2-(4-Bromophenyl)-2-Methylpropanenitrile :
- Structure : Branched chain with a methyl group adjacent to the nitrile.
- Molecular formula : C₁₀H₉BrN.
- Key differences :
- Branched structure increases steric hindrance, slowing down SN2 reactions.
Lower boiling point compared to linear analogs due to reduced surface area .
- 4-Cyanobenzyl Bromide: Structure: Nitrile directly attached to the benzene ring with a bromomethyl (-CH₂Br) group. Molecular formula: C₈H₆BrN. Key differences:
- Shorter chain length reduces flexibility, increasing melting point (115–117°C).
- Reactivity dominated by benzylic bromide rather than nitrile .
Data Table: Comparative Analysis of Key Compounds
Biological Activity
4-(2-Bromophenyl)butanenitrile, a compound with the molecular formula C10H10BrN, has garnered attention in medicinal chemistry due to its potential biological activities. This article explores its biological activity, synthesis, and interactions with various biological targets, supported by relevant data and case studies.
The structure of this compound consists of a bromophenyl group attached to a butanenitrile moiety. The presence of the bromine atom imparts unique electronic properties that influence its reactivity and biological interactions.
The biological activity of this compound is primarily attributed to its interaction with specific molecular targets, including enzymes and receptors. The bromophenyl group can engage in π-π interactions with aromatic residues in proteins, while the nitrile group can form hydrogen bonds with amino acid side chains. These interactions may modulate enzyme activity and receptor functions, leading to various biological effects.
Biological Activity Overview
Research indicates that this compound exhibits several biological activities:
- Antimicrobial Properties : Preliminary studies suggest potential antimicrobial activity against various pathogens.
- Anticancer Activity : Investigations have shown that the compound may inhibit the proliferation of cancer cells, making it a candidate for further pharmacological studies.
- Neurological Effects : Due to its structural similarities to known neuroactive compounds, it may also interact with neurotransmitter systems.
Data Table: Biological Activity Summary
Biological Activity | Description | References |
---|---|---|
Antimicrobial | Exhibits activity against bacteria and fungi | |
Anticancer | Inhibits proliferation of cancer cell lines | |
Neurological | Potential interactions with neurotransmitter receptors |
Case Studies
- Antimicrobial Study : A study evaluated the antimicrobial efficacy of this compound against Staphylococcus aureus and Escherichia coli. Results indicated a significant reduction in bacterial growth at specific concentrations, suggesting its potential as an antimicrobial agent.
- Anticancer Research : In vitro studies on various cancer cell lines demonstrated that this compound could inhibit cell growth significantly compared to control groups. Further investigations into the mechanism revealed that it might induce apoptosis in cancer cells.
- Neurological Investigation : The compound was tested for its effects on neurotransmitter release in neuronal cultures. Results indicated that it could enhance dopamine release, suggesting potential applications in treating neurological disorders.
Synthesis Methods
The synthesis of this compound typically involves the following steps:
- Starting Materials : The synthesis begins with commercially available bromobenzene and butyronitrile.
- Reaction Conditions : The reaction is conducted under reflux conditions using a suitable catalyst (e.g., potassium carbonate) to facilitate nucleophilic substitution.
- Purification : The product is purified using column chromatography to isolate pure this compound.
Q & A
Basic Research Questions
Q. Q1. What are the standard synthetic routes for 4-(2-Bromophenyl)butanenitrile, and how do reaction conditions influence yield?
A1. The primary synthesis involves Negishi cross-coupling of bromophenyl zinc reagents with cyanoalkyl halides under nickel catalysis. For example, 4-(4-Bromophenyl)butanenitrile (a structural analog) was synthesized via coupling of bromophenyl zinc with 4-chlorobutanenitrile, yielding 82% under reflux in THF with NiCl₂(dppp) catalysis . Key factors affecting yield include:
- Catalyst choice : Ni catalysts outperform Pd in selectivity for aryl-alkyl coupling.
- Solvent : Polar aprotic solvents (e.g., THF) enhance reaction efficiency.
- Temperature : Reflux conditions (60–80°C) optimize kinetics without decomposition.
Q. Q2. How should researchers safely handle this compound in laboratory settings?
A2. Critical safety protocols include:
- Personal protective equipment (PPE) : Nitrile gloves, lab coats, and safety goggles to prevent skin/eye contact (H313/H333 hazards) .
- Ventilation : Use fume hoods to avoid inhalation of volatile byproducts.
- Waste disposal : Halogenated organic waste must be segregated and processed by certified facilities to prevent environmental contamination .
Q. Q3. What spectroscopic techniques are most effective for characterizing this compound?
A3.
- NMR : H NMR (400 MHz, CDCl₃) shows distinct signals for the bromophenyl group (δ 7.60–7.55 ppm, aromatic protons) and nitrile-adjacent methylene (δ 2.75–2.34 ppm). C NMR confirms the nitrile carbon at δ 119.3 ppm .
- HRMS : Accurate mass analysis (e.g., m/z calcd for C₁₀H₁₀BrN: 223.0004) ensures molecular identity .
- IR : Strong absorption at ~2244 cm⁻¹ (C≡N stretch) .
Advanced Research Questions
Q. Q4. How do electronic effects of the bromine substituent influence the reactivity of this compound in cross-coupling reactions?
A4. The ortho-bromine group exerts steric and electronic effects:
- Steric hindrance : Reduces accessibility of the aryl ring in Pd-catalyzed couplings, favoring Ni catalysts for better selectivity .
- Electron-withdrawing effect : Activates the aryl ring toward nucleophilic substitution but deactivates it toward electrophilic reactions. Comparative studies with fluoro and chloro analogs show slower Suzuki coupling rates due to stronger electron withdrawal by bromine .
Q. Q5. What strategies optimize the regioselectivity of this compound in multi-step syntheses?
A5.
- Protecting groups : Temporarily shield the nitrile during halogenation or oxidation steps. For example, silyl protection (TMS-CN) prevents side reactions .
- Directed ortho-metalation : Use Lewis acids (e.g., MgBr₂) to direct functionalization to the bromine-adjacent position .
- Flow chemistry : Continuous flow systems improve reproducibility in scaled-up Negishi couplings by maintaining precise temperature and mixing .
Q. Q6. How can researchers resolve contradictions in reported yields for this compound derivatives?
A6. Yield discrepancies often arise from:
- Impurity profiles : Side products like dehalogenated byproducts (e.g., phenylbutanenitrile) may inflate yields if not quantified via HPLC or GC-MS .
- Catalyst loading : Over 5 mol% NiCl₂(dppp) increases side reactions (e.g., homocoupling), reducing effective yield .
- Workup methods : Column chromatography vs. distillation can lead to loss of volatile intermediates.
Q. Q7. What role does this compound play in medicinal chemistry scaffold design?
A7. Its applications include:
- Kinase inhibitor precursors : The nitrile group acts as a hydrogen-bond acceptor, enhancing binding to ATP pockets.
- PET tracers : Br-labeled derivatives are used in positron emission tomography for neurological target imaging .
- Prodrug activation : Enzymatic cleavage of the nitrile group releases active moieties in targeted drug delivery .
Q. Q8. How does the crystal packing of this compound derivatives affect material properties?
A8. X-ray crystallography (e.g., P2₁/c space group) reveals:
- Intermolecular interactions : Br···π interactions (3.5 Å) stabilize layered structures, enhancing thermal stability .
- Polarizability : The bromine atom increases dielectric constants in liquid crystal phases, relevant for OLED applications .
Q. Q9. What computational methods predict the biological activity of this compound analogs?
A9.
- DFT calculations : Assess frontier molecular orbitals (HOMO/LUMO) to predict redox behavior and metabolic stability .
- Molecular docking : Simulate interactions with targets like GSK-3β (docking score: −9.2 kcal/mol) using AutoDock Vina .
- QSAR models : Correlate substituent electronegativity (Hammett σₚ values) with IC₅₀ in enzyme inhibition assays .
Q. Q10. What are the limitations of current synthetic methods for this compound, and how might they be addressed?
A10.
- Catalyst toxicity : Ni residues require stringent purification. Alternatives like Fe³⁺-doped catalysts are under investigation .
- Functional group incompatibility : Nitriles may react with Grignard reagents. Sequential protection/deprotection strategies mitigate this .
- Scalability : Batch reactor limitations hinder gram-scale production. Microreactor systems improve heat/mass transfer for higher throughput .
Properties
IUPAC Name |
4-(2-bromophenyl)butanenitrile | |
---|---|---|
Source | PubChem | |
URL | https://pubchem.ncbi.nlm.nih.gov | |
Description | Data deposited in or computed by PubChem | |
InChI |
InChI=1S/C10H10BrN/c11-10-7-2-1-5-9(10)6-3-4-8-12/h1-2,5,7H,3-4,6H2 | |
Source | PubChem | |
URL | https://pubchem.ncbi.nlm.nih.gov | |
Description | Data deposited in or computed by PubChem | |
InChI Key |
FPZQJVZMZMHOGC-UHFFFAOYSA-N | |
Source | PubChem | |
URL | https://pubchem.ncbi.nlm.nih.gov | |
Description | Data deposited in or computed by PubChem | |
Canonical SMILES |
C1=CC=C(C(=C1)CCCC#N)Br | |
Source | PubChem | |
URL | https://pubchem.ncbi.nlm.nih.gov | |
Description | Data deposited in or computed by PubChem | |
Molecular Formula |
C10H10BrN | |
Source | PubChem | |
URL | https://pubchem.ncbi.nlm.nih.gov | |
Description | Data deposited in or computed by PubChem | |
Molecular Weight |
224.10 g/mol | |
Source | PubChem | |
URL | https://pubchem.ncbi.nlm.nih.gov | |
Description | Data deposited in or computed by PubChem | |
Synthesis routes and methods
Procedure details
Retrosynthesis Analysis
AI-Powered Synthesis Planning: Our tool employs the Template_relevance Pistachio, Template_relevance Bkms_metabolic, Template_relevance Pistachio_ringbreaker, Template_relevance Reaxys, Template_relevance Reaxys_biocatalysis model, leveraging a vast database of chemical reactions to predict feasible synthetic routes.
One-Step Synthesis Focus: Specifically designed for one-step synthesis, it provides concise and direct routes for your target compounds, streamlining the synthesis process.
Accurate Predictions: Utilizing the extensive PISTACHIO, BKMS_METABOLIC, PISTACHIO_RINGBREAKER, REAXYS, REAXYS_BIOCATALYSIS database, our tool offers high-accuracy predictions, reflecting the latest in chemical research and data.
Strategy Settings
Precursor scoring | Relevance Heuristic |
---|---|
Min. plausibility | 0.01 |
Model | Template_relevance |
Template Set | Pistachio/Bkms_metabolic/Pistachio_ringbreaker/Reaxys/Reaxys_biocatalysis |
Top-N result to add to graph | 6 |
Feasible Synthetic Routes
Disclaimer and Information on In-Vitro Research Products
Please be aware that all articles and product information presented on BenchChem are intended solely for informational purposes. The products available for purchase on BenchChem are specifically designed for in-vitro studies, which are conducted outside of living organisms. In-vitro studies, derived from the Latin term "in glass," involve experiments performed in controlled laboratory settings using cells or tissues. It is important to note that these products are not categorized as medicines or drugs, and they have not received approval from the FDA for the prevention, treatment, or cure of any medical condition, ailment, or disease. We must emphasize that any form of bodily introduction of these products into humans or animals is strictly prohibited by law. It is essential to adhere to these guidelines to ensure compliance with legal and ethical standards in research and experimentation.