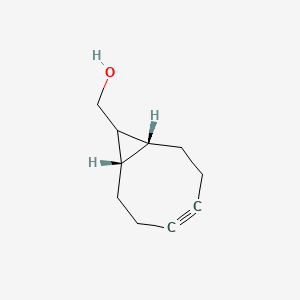
Bcn-OH
Overview
Description
Bcn-OH, also known as endo-9-Hydroxymethylbicyclo[6.1.0]non-4-yne, is a compound that serves as a mitochondrial probe based on the lyophilic bidentate bicyclic ligand BCN. It is often used as a control reagent for BCN-TPP, a reactive sulfenic acid probe that targets mitochondria .
Biochemical Analysis
Biochemical Properties
Bcn-OH plays a significant role in biochemical reactions. It reacts with chemicals or biomolecules that have azide functionalization to produce a stable triazole bond . This reaction occurs without the need for a copper catalyst .
Cellular Effects
It is known that BCN-TPP, a related compound, affects mitochondrial energy, causing a sharp decrease in basal respiration .
Molecular Mechanism
This compound exerts its effects at the molecular level through its interactions with other biomolecules. It forms a stable triazole bond with azide-functionalized chemicals or biomolecules .
Preparation Methods
The synthesis of Bcn-OH involves the use of boric acid and hexamethylenetetramine as boron and carbon sources, respectively. The compound is synthesized using melamine and guanidine hydrochloride as the nitrogen source . The preparation process includes thermal annealing at 800°C, which results in the formation of BCN-doped hexagonal boron nitride nanodisks . Industrial production methods typically involve similar synthetic routes but on a larger scale, ensuring high purity and yield.
Chemical Reactions Analysis
Bcn-OH undergoes various types of chemical reactions, including oxidation, reduction, and substitution. Common reagents used in these reactions include azide-tagged molecules or biomolecules, which react with the BCN group via copper-free Click Chemistry . The major products formed from these reactions are triazole compounds, which are useful in bioconjugation and labeling applications .
Scientific Research Applications
Bcn-OH has a wide range of scientific research applications. In chemistry, it is used as a probe for studying mitochondrial function and energy metabolism . In biology, it serves as a tool for labeling and tracking biomolecules in live cells . In medicine, this compound is used in drug delivery systems and diagnostics due to its ability to target specific cellular components . Industrial applications include its use in the development of novel materials and nanostructures .
Mechanism of Action
The mechanism of action of Bcn-OH involves its interaction with mitochondrial proteins. The compound affects mitochondrial energy by causing a sharp decrease in basal respiration, which is attributed to its faster reaction kinetics with sulfonated proteins . This interaction allows researchers to study mitochondrial function and identify potential therapeutic targets.
Comparison with Similar Compounds
Bcn-OH is unique compared to other similar compounds due to its specific interaction with mitochondrial proteins and its use as a control reagent for BCN-TPP . Similar compounds include other BCN-based probes and reagents, such as endo-BCN-PEG-alcohol and endo-BCN-PEG-amine . These compounds share similar chemical structures but differ in their specific applications and reactivity.
Properties
IUPAC Name |
[(1S,8R)-9-bicyclo[6.1.0]non-4-ynyl]methanol | |
---|---|---|
Source | PubChem | |
URL | https://pubchem.ncbi.nlm.nih.gov | |
Description | Data deposited in or computed by PubChem | |
InChI |
InChI=1S/C10H14O/c11-7-10-8-5-3-1-2-4-6-9(8)10/h8-11H,3-7H2/t8-,9+,10? | |
Source | PubChem | |
URL | https://pubchem.ncbi.nlm.nih.gov | |
Description | Data deposited in or computed by PubChem | |
InChI Key |
NSVXZMGWYBICRW-ULKQDVFKSA-N | |
Source | PubChem | |
URL | https://pubchem.ncbi.nlm.nih.gov | |
Description | Data deposited in or computed by PubChem | |
Canonical SMILES |
C1CC2C(C2CO)CCC#C1 | |
Source | PubChem | |
URL | https://pubchem.ncbi.nlm.nih.gov | |
Description | Data deposited in or computed by PubChem | |
Isomeric SMILES |
C1C[C@@H]2[C@@H](C2CO)CCC#C1 | |
Source | PubChem | |
URL | https://pubchem.ncbi.nlm.nih.gov | |
Description | Data deposited in or computed by PubChem | |
Molecular Formula |
C10H14O | |
Source | PubChem | |
URL | https://pubchem.ncbi.nlm.nih.gov | |
Description | Data deposited in or computed by PubChem | |
DSSTOX Substance ID |
DTXSID601254298 | |
Record name | (1α,8α,9α)-Bicyclo[6.1.0]non-4-yne-9-methanol | |
Source | EPA DSSTox | |
URL | https://comptox.epa.gov/dashboard/DTXSID601254298 | |
Description | DSSTox provides a high quality public chemistry resource for supporting improved predictive toxicology. | |
Molecular Weight |
150.22 g/mol | |
Source | PubChem | |
URL | https://pubchem.ncbi.nlm.nih.gov | |
Description | Data deposited in or computed by PubChem | |
CAS No. |
1263291-41-3 | |
Record name | (1α,8α,9α)-Bicyclo[6.1.0]non-4-yne-9-methanol | |
Source | EPA DSSTox | |
URL | https://comptox.epa.gov/dashboard/DTXSID601254298 | |
Description | DSSTox provides a high quality public chemistry resource for supporting improved predictive toxicology. | |
Retrosynthesis Analysis
AI-Powered Synthesis Planning: Our tool employs the Template_relevance Pistachio, Template_relevance Bkms_metabolic, Template_relevance Pistachio_ringbreaker, Template_relevance Reaxys, Template_relevance Reaxys_biocatalysis model, leveraging a vast database of chemical reactions to predict feasible synthetic routes.
One-Step Synthesis Focus: Specifically designed for one-step synthesis, it provides concise and direct routes for your target compounds, streamlining the synthesis process.
Accurate Predictions: Utilizing the extensive PISTACHIO, BKMS_METABOLIC, PISTACHIO_RINGBREAKER, REAXYS, REAXYS_BIOCATALYSIS database, our tool offers high-accuracy predictions, reflecting the latest in chemical research and data.
Strategy Settings
Precursor scoring | Relevance Heuristic |
---|---|
Min. plausibility | 0.01 |
Model | Template_relevance |
Template Set | Pistachio/Bkms_metabolic/Pistachio_ringbreaker/Reaxys/Reaxys_biocatalysis |
Top-N result to add to graph | 6 |
Feasible Synthetic Routes
Q1: How does BCN-OH interact with its target, and what are the downstream effects?
A1: this compound reacts specifically with azide groups, typically introduced into biomolecules through metabolic labeling or other modifications. This reaction, known as SPAAC, forms a stable triazole linkage. [, , , ] This bioorthogonal reaction proceeds rapidly under physiological conditions without requiring toxic catalysts, making it valuable for live-cell labeling and imaging. [, ] For example, this compound conjugated to a phosphorogenic rhenium(I) complex enabled specific lysosome labeling in live cells. [] Another study demonstrated the use of a this compound-based "click-and-release" system for intracellular delivery of carbon disulfide (CS2), allowing investigation of CS2-induced hepatotoxicity. []
Q2: What is the reactivity difference between this compound and other strained alkynes/alkenes in SPAAC reactions?
A2: Research has shown that this compound reacts much faster with 1,2-quinone in a SPAAC reaction compared to the strained alkene TCO-OH. [] Interestingly, while an 8-membered ring, this compound reacts faster than the more strained 7-membered alkyne THS in SPAAC reactions with 1,2-quinone. Thermodynamic analysis suggests that this difference in reaction rate is mainly dictated by entropic factors. []
Q3: Are there any studies investigating the impact of the triphenylphosphonium (TPP) group on this compound's reactivity?
A3: Yes, researchers have explored the use of TPP as a mitochondrial targeting group for this compound. When linked to this compound, the resulting BCN-TPP conjugate showed a 4.6-fold decrease in reaction rate with a model protein sulfenic acid compared to this compound alone. [] This suggests potential steric hindrance from the TPP group affecting the optimal reaction orientation. Interestingly, BCN-TPP, unlike this compound, did not react with protein persulfides, highlighting its potential selectivity. []
Disclaimer and Information on In-Vitro Research Products
Please be aware that all articles and product information presented on BenchChem are intended solely for informational purposes. The products available for purchase on BenchChem are specifically designed for in-vitro studies, which are conducted outside of living organisms. In-vitro studies, derived from the Latin term "in glass," involve experiments performed in controlled laboratory settings using cells or tissues. It is important to note that these products are not categorized as medicines or drugs, and they have not received approval from the FDA for the prevention, treatment, or cure of any medical condition, ailment, or disease. We must emphasize that any form of bodily introduction of these products into humans or animals is strictly prohibited by law. It is essential to adhere to these guidelines to ensure compliance with legal and ethical standards in research and experimentation.