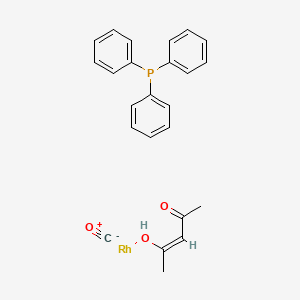
Rhodium (triphenylphosphine)carbonylacetylacetonate
- Click on QUICK INQUIRY to receive a quote from our team of experts.
- With the quality product at a COMPETITIVE price, you can focus more on your research.
Overview
Description
Rhodium (triphenylphosphine)carbonylacetylacetonate: is a coordination complex with the molecular formula C24H23O3PRh and a molecular weight of 493.32 g/mol . This compound is known for its yellow color and solid form. It is soluble in acetone and chlorinated solvents . This compound is widely used as a catalyst in various chemical reactions, particularly in the petrochemical industry .
Preparation Methods
Synthetic Routes and Reaction Conditions: The synthesis of Rhodium (triphenylphosphine)carbonylacetylacetonate typically involves the reaction of rhodium chloride trihydrate with triphenylphosphine in the presence of N,N-dimethylformamide under nitrogen protection . The mixture is then heated under reflux, followed by the addition of acetylacetone. After cooling to room temperature, the solution is concentrated, and the product is precipitated by adding icy water. The resulting crystals are filtered, washed with water, and vacuum-dried to obtain the final product .
Industrial Production Methods: Industrial production of this compound follows similar synthetic routes but on a larger scale. The process parameters, such as reaction temperature, reaction time, and phase ratio, are optimized to maximize yield and efficiency .
Chemical Reactions Analysis
Types of Reactions: Rhodium (triphenylphosphine)carbonylacetylacetonate undergoes various chemical reactions, including:
Oxidation: The compound can be oxidized to form rhodium(III) complexes.
Reduction: It can be reduced to lower oxidation states of rhodium.
Substitution: The triphenylphosphine ligand can be substituted with other ligands under appropriate conditions.
Common Reagents and Conditions:
Oxidation: Hydrogen peroxide (H2O2) is commonly used as an oxidizing agent.
Reduction: Reducing agents such as hydrogen gas (H2) or sodium borohydride (NaBH4) are used.
Substitution: Ligand exchange reactions often involve the use of other phosphine ligands or nitrogen-based ligands.
Major Products:
Oxidation: Rhodium(III) complexes such as chloroaquorhodium(III) complexes.
Reduction: Lower oxidation state rhodium complexes.
Substitution: New rhodium complexes with different ligands.
Scientific Research Applications
Rhodium (triphenylphosphine)carbonylacetylacetonate has a wide range of applications in scientific research, including:
Mechanism of Action
The mechanism of action of Rhodium (triphenylphosphine)carbonylacetylacetonate involves the coordination of the rhodium center with the ligands (triphenylphosphine, carbonyl, and acetylacetonate). This coordination facilitates various catalytic reactions by stabilizing transition states and intermediates . The molecular targets and pathways involved depend on the specific reaction being catalyzed. For example, in hydroformylation, the rhodium center coordinates with the alkene substrate, facilitating the addition of a formyl group to produce aldehydes .
Comparison with Similar Compounds
Rhodium acetylacetonate (Rh(acac)3): This compound has a similar coordination environment but lacks the triphenylphosphine and carbonyl ligands.
Dicarbonyl (acetylacetonato)rhodium(I) (Rh(acac)(CO)2): This compound contains two carbonyl ligands and one acetylacetonate ligand.
Iridium acetylacetonate (Ir(acac)3): Similar to rhodium acetylacetonate but with iridium as the central metal.
Uniqueness: Rhodium (triphenylphosphine)carbonylacetylacetonate is unique due to the presence of the triphenylphosphine ligand, which enhances its catalytic properties and stability . This makes it particularly effective in hydroformylation reactions and other catalytic processes .
Properties
Molecular Formula |
C24H23O3PRh |
---|---|
Molecular Weight |
493.3 g/mol |
IUPAC Name |
carbon monoxide;(Z)-4-hydroxypent-3-en-2-one;rhodium;triphenylphosphane |
InChI |
InChI=1S/C18H15P.C5H8O2.CO.Rh/c1-4-10-16(11-5-1)19(17-12-6-2-7-13-17)18-14-8-3-9-15-18;1-4(6)3-5(2)7;1-2;/h1-15H;3,6H,1-2H3;;/b;4-3-;; |
InChI Key |
RHKGZYVYKXVQSD-MECAPONASA-N |
Isomeric SMILES |
C/C(=C/C(=O)C)/O.[C-]#[O+].C1=CC=C(C=C1)P(C2=CC=CC=C2)C3=CC=CC=C3.[Rh] |
Canonical SMILES |
CC(=CC(=O)C)O.[C-]#[O+].C1=CC=C(C=C1)P(C2=CC=CC=C2)C3=CC=CC=C3.[Rh] |
Origin of Product |
United States |
Disclaimer and Information on In-Vitro Research Products
Please be aware that all articles and product information presented on BenchChem are intended solely for informational purposes. The products available for purchase on BenchChem are specifically designed for in-vitro studies, which are conducted outside of living organisms. In-vitro studies, derived from the Latin term "in glass," involve experiments performed in controlled laboratory settings using cells or tissues. It is important to note that these products are not categorized as medicines or drugs, and they have not received approval from the FDA for the prevention, treatment, or cure of any medical condition, ailment, or disease. We must emphasize that any form of bodily introduction of these products into humans or animals is strictly prohibited by law. It is essential to adhere to these guidelines to ensure compliance with legal and ethical standards in research and experimentation.