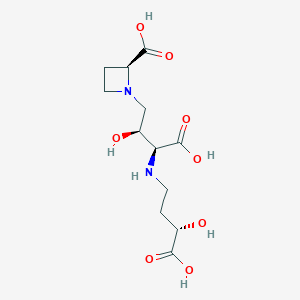
Mugineic acid
Overview
Description
Mugineic acid is an organic compound characterized by an azetidine group and three carboxylate groups. It is a colorless solid and functions as a siderophore, specifically a phytosiderophore, which is a plant-produced siderophore. This compound plays a crucial role in iron accumulation for barley and other plants . It is biosynthesized from S-methylmethionine and binds metal ions as a hexadentate ligand .
Preparation Methods
Synthetic Routes and Reaction Conditions: The total synthesis of mugineic acid involves a series of steps starting from chiral pool starting materials such as L-malic acid and threonines. The synthesis includes diastereo- and enantioselective methods to prepare the middle building blocks. A significant highlight of this synthesis is the preparation of epimeric hydroxyazetidine amino acids, which are useful building blocks .
Industrial Production Methods: Industrial production methods for this compound are not extensively documented. the synthesis of related compounds like 2’-deoxythis compound involves practical synthesis and transport of its iron-chelated form across the plasma membrane by specific transporters .
Chemical Reactions Analysis
Types of Reactions: Mugineic acid undergoes various chemical reactions, including complexation with metal ions. It forms stable complexes with iron (III) ions, which are crucial for its function as a phytosiderophore .
Common Reagents and Conditions: The complexation reactions typically involve iron (III) ions and occur under conditions that facilitate the formation of stable 1:1 complexes. The coordination involves the azetidine nitrogen, secondary amine nitrogen, terminal carboxylate oxygens, hydroxyl oxygen, and intermediate carboxylate oxygen .
Major Products: The major products of these reactions are the iron (III)-mugineic acid complexes, which are essential for iron uptake and transport in plants .
Scientific Research Applications
Mugineic acid has several scientific research applications, particularly in the fields of agriculture and plant biology. It is used to enhance iron uptake in plants, especially in calcareous soils where iron availability is limited. The application of synthetic this compound analogs has shown promise in recovering iron deficiency in crops like rice . Additionally, this compound and its analogs are being explored as iron fertilizers to improve agricultural productivity .
Mechanism of Action
Mugineic acid functions by chelating iron (III) ions in the soil, forming a stable complex that plants can absorb. The coordination involves multiple ligand functional groups, including carboxyl, amine, and hydroxyl groups . The iron (III)-mugineic acid complex is then transported into plant cells via specific transporters, where the iron is released and utilized for various physiological processes .
Comparison with Similar Compounds
Epi-hydroxymugineic acid: Similar to this compound but with a hydroxyl group at a different position.
Nicotianamine: A related compound that binds iron (II), copper (II), and zinc (II) and plays a role in long-distance metal transport in plants.
Uniqueness: this compound is unique due to its specific role as a phytosiderophore in gramineous plants. It has a high affinity for iron (III) ions, which makes it particularly effective in iron uptake under alkaline soil conditions where iron availability is limited .
Properties
IUPAC Name |
1-[3-carboxy-3-[(3-carboxy-3-hydroxypropyl)amino]-2-hydroxypropyl]azetidine-2-carboxylic acid | |
---|---|---|
Details | Computed by LexiChem 2.6.6 (PubChem release 2019.06.18) | |
Source | PubChem | |
URL | https://pubchem.ncbi.nlm.nih.gov | |
Description | Data deposited in or computed by PubChem | |
InChI |
InChI=1S/C12H20N2O8/c15-7(11(19)20)1-3-13-9(12(21)22)8(16)5-14-4-2-6(14)10(17)18/h6-9,13,15-16H,1-5H2,(H,17,18)(H,19,20)(H,21,22) | |
Details | Computed by InChI 1.0.5 (PubChem release 2019.06.18) | |
Source | PubChem | |
URL | https://pubchem.ncbi.nlm.nih.gov | |
Description | Data deposited in or computed by PubChem | |
InChI Key |
GJRGEVKCJPPZIT-UHFFFAOYSA-N | |
Details | Computed by InChI 1.0.5 (PubChem release 2019.06.18) | |
Source | PubChem | |
URL | https://pubchem.ncbi.nlm.nih.gov | |
Description | Data deposited in or computed by PubChem | |
Canonical SMILES |
C1CN(C1C(=O)O)CC(C(C(=O)O)NCCC(C(=O)O)O)O | |
Details | Computed by OEChem 2.1.5 (PubChem release 2019.06.18) | |
Source | PubChem | |
URL | https://pubchem.ncbi.nlm.nih.gov | |
Description | Data deposited in or computed by PubChem | |
Molecular Formula |
C12H20N2O8 | |
Details | Computed by PubChem 2.1 (PubChem release 2019.06.18) | |
Source | PubChem | |
URL | https://pubchem.ncbi.nlm.nih.gov | |
Description | Data deposited in or computed by PubChem | |
DSSTOX Substance ID |
DTXSID90988904 | |
Record name | Mugineic_acid | |
Source | EPA DSSTox | |
URL | https://comptox.epa.gov/dashboard/DTXSID90988904 | |
Description | DSSTox provides a high quality public chemistry resource for supporting improved predictive toxicology. | |
Molecular Weight |
320.30 g/mol | |
Details | Computed by PubChem 2.1 (PubChem release 2021.05.07) | |
Source | PubChem | |
URL | https://pubchem.ncbi.nlm.nih.gov | |
Description | Data deposited in or computed by PubChem | |
CAS No. |
69199-37-7, 74281-81-5 | |
Record name | Mugineic_acid | |
Source | EPA DSSTox | |
URL | https://comptox.epa.gov/dashboard/DTXSID90988904 | |
Description | DSSTox provides a high quality public chemistry resource for supporting improved predictive toxicology. | |
Record name | Isomugineic acid | |
Source | Human Metabolome Database (HMDB) | |
URL | http://www.hmdb.ca/metabolites/HMDB0033555 | |
Description | The Human Metabolome Database (HMDB) is a freely available electronic database containing detailed information about small molecule metabolites found in the human body. | |
Explanation | HMDB is offered to the public as a freely available resource. Use and re-distribution of the data, in whole or in part, for commercial purposes requires explicit permission of the authors and explicit acknowledgment of the source material (HMDB) and the original publication (see the HMDB citing page). We ask that users who download significant portions of the database cite the HMDB paper in any resulting publications. | |
Retrosynthesis Analysis
AI-Powered Synthesis Planning: Our tool employs the Template_relevance Pistachio, Template_relevance Bkms_metabolic, Template_relevance Pistachio_ringbreaker, Template_relevance Reaxys, Template_relevance Reaxys_biocatalysis model, leveraging a vast database of chemical reactions to predict feasible synthetic routes.
One-Step Synthesis Focus: Specifically designed for one-step synthesis, it provides concise and direct routes for your target compounds, streamlining the synthesis process.
Accurate Predictions: Utilizing the extensive PISTACHIO, BKMS_METABOLIC, PISTACHIO_RINGBREAKER, REAXYS, REAXYS_BIOCATALYSIS database, our tool offers high-accuracy predictions, reflecting the latest in chemical research and data.
Strategy Settings
Precursor scoring | Relevance Heuristic |
---|---|
Min. plausibility | 0.01 |
Model | Template_relevance |
Template Set | Pistachio/Bkms_metabolic/Pistachio_ringbreaker/Reaxys/Reaxys_biocatalysis |
Top-N result to add to graph | 6 |
Feasible Synthetic Routes
Disclaimer and Information on In-Vitro Research Products
Please be aware that all articles and product information presented on BenchChem are intended solely for informational purposes. The products available for purchase on BenchChem are specifically designed for in-vitro studies, which are conducted outside of living organisms. In-vitro studies, derived from the Latin term "in glass," involve experiments performed in controlled laboratory settings using cells or tissues. It is important to note that these products are not categorized as medicines or drugs, and they have not received approval from the FDA for the prevention, treatment, or cure of any medical condition, ailment, or disease. We must emphasize that any form of bodily introduction of these products into humans or animals is strictly prohibited by law. It is essential to adhere to these guidelines to ensure compliance with legal and ethical standards in research and experimentation.