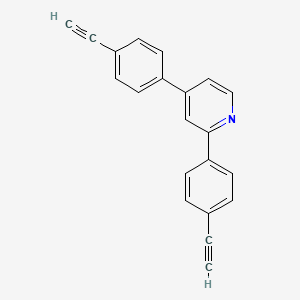
2,4-Bis(4-ethynylphenyl)pyridine
- Click on QUICK INQUIRY to receive a quote from our team of experts.
- With the quality product at a COMPETITIVE price, you can focus more on your research.
Overview
Description
2,4-Bis(4-ethynylphenyl)pyridine is a pyridine-based compound featuring two ethynylphenyl substituents at the 2- and 4-positions of the pyridine ring. The ethynyl (-C≡C-) groups confer rigidity and extended π-conjugation, making it a candidate for applications in optoelectronics and coordination chemistry. For instance, substituent effects on melting points, solubility, and electronic behavior can be inferred from similar compounds .
Preparation Methods
Synthetic Routes and Reaction Conditions: The synthesis of 2,4-Bis(4-ethynylphenyl)pyridine typically involves the coupling of pyridine derivatives with ethynylbenzene derivatives. One common method is the palladium-catalyzed cross-coupling reaction, such as the Suzuki or Sonogashira coupling . These reactions are carried out under inert conditions, often using solvents like tetrahydrofuran (THF) or dimethylformamide (DMF), and require the presence of a base such as potassium carbonate (K2CO3) or triethylamine (TEA).
Industrial Production Methods: Industrial production of this compound may involve similar coupling reactions on a larger scale, with optimization for yield and purity. The use of continuous flow reactors and advanced purification techniques, such as column chromatography, can enhance the efficiency of the production process .
Chemical Reactions Analysis
Types of Reactions: 2,4-Bis(4-ethynylphenyl)pyridine can undergo various chemical reactions, including:
Oxidation: The ethynyl groups can be oxidized to form carbonyl compounds.
Reduction: The pyridine ring can be reduced to form piperidine derivatives.
Substitution: The ethynyl groups can participate in nucleophilic substitution reactions.
Common Reagents and Conditions:
Oxidation: Reagents such as potassium permanganate (KMnO4) or chromium trioxide (CrO3) in acidic conditions.
Reduction: Hydrogen gas (H2) in the presence of a palladium catalyst (Pd/C).
Substitution: Nucleophiles like sodium azide (NaN3) or lithium aluminum hydride (LiAlH4) under appropriate conditions.
Major Products:
Oxidation: Formation of diketones or carboxylic acids.
Reduction: Formation of piperidine derivatives.
Substitution: Formation of substituted pyridine derivatives.
Scientific Research Applications
2,4-Bis(4-ethynylphenyl)pyridine applications in scientific research:
This compound is a chemical compound with the molecular formula C21H13N and a molecular weight of 279.33 . It belongs to the class of pyridine derivatives, which have a wide range of applications in various scientific fields .
Scientific Research Applications
- Synthesis of complex organic molecules Pyridine derivatives are used as intermediates in the synthesis of complex organic molecules, including pharmaceuticals and agrochemicals.
- Study of biological activities Derivatives of pyridine are studied for their potential biological activities, such as enzyme inhibition.
- Antimicrobial activity Pyridine compounds have demonstrated antimicrobial activities against various strains of bacteria, yeasts, and fungi .
- Anticancer agents Pyridine derivatives have attracted interest for their anticancer activities, with numerous analogs in development or approved for cancer treatment .
- Material Science Pyridine and its derivatives are also used as a building block in material science. For example, it is used in the design of molecular architectures .
Documents
this compound information includes NMR, HPLC, LC-MS and UPLC .
Mechanism of Action
The mechanism of action of 2,4-Bis(4-ethynylphenyl)pyridine involves its interaction with specific molecular targets and pathways. In biological systems, it may act as a ligand that binds to metal ions or proteins, modulating their activity. The ethynyl groups can participate in π-π interactions and hydrogen bonding, influencing the compound’s binding affinity and specificity .
Comparison with Similar Compounds
Substituent Effects on Physical Properties
The substituents on pyridine derivatives significantly influence their physical and chemical properties. Below is a comparison with structurally related compounds:
*Estimated based on molecular formula.
†Molecular weight inferred from complex structure.
Key Observations:
- Electronic Effects: Ethynyl groups are electron-withdrawing via conjugation, contrasting with electron-donating groups (e.g., -OCH₃) in PDCA or halogenated derivatives (e.g., -Cl) in compounds. This impacts reactivity and optoelectronic behavior .
- Thermal Stability: Halogenated pyridines (e.g., Cl-substituted) exhibit higher melting points (~287°C) due to intermolecular halogen bonding, whereas ethynyl-substituted derivatives may prioritize thermal stability from rigid conjugation .
Research Findings on Substituent Impact
- Solubility: Alkyl-substituted pyridines (e.g., -CH₃) in show moderate solubility in organic solvents, while ethynyl groups may reduce solubility due to increased hydrophobicity .
- Biological Interactions: PDCA reduces hydroxyproline content in tomato roots by >70% at 250 µM, highlighting the role of carboxylic acid groups in enzyme inhibition. Ethynyl-substituted analogs lack such functional moieties, limiting bioactivity .
- Optoelectronic Performance: Iridium complexes with difluorophenylpyridine ligands (e.g., Ir-D1) achieve high quantum yields (~80%), suggesting that ethynyl groups could similarly enhance luminescence efficiency via conjugation .
Biological Activity
2,4-Bis(4-ethynylphenyl)pyridine (BEPP) is a pyridine derivative that has garnered attention for its potential biological activities. This compound features a unique structure that allows it to interact with various biological targets, making it a subject of interest in medicinal chemistry and pharmacology. This article provides a comprehensive overview of the biological activity of BEPP, including its mechanisms of action, therapeutic potential, and relevant case studies.
Chemical Structure and Properties
The molecular formula of this compound is C18H14N, with a molecular weight of 250.32 g/mol. The compound consists of a pyridine ring substituted with two ethynylphenyl groups at the 2 and 4 positions.
Property | Value |
---|---|
Molecular Formula | C18H14N |
Molecular Weight | 250.32 g/mol |
IUPAC Name | This compound |
The biological activity of BEPP is primarily attributed to its ability to interact with specific enzymes and receptors within biological systems. Research suggests that BEPP may act as an enzyme inhibitor or modulator, affecting pathways related to cell signaling and proliferation.
- Enzyme Inhibition : BEPP has been shown to inhibit certain enzymes involved in metabolic pathways, which can lead to altered cellular functions.
- Receptor Interaction : The compound may bind to specific receptors, modulating their activity and influencing downstream signaling cascades.
Biological Activities
Recent studies have explored the diverse biological activities associated with BEPP:
- Anticancer Activity : BEPP has demonstrated cytotoxic effects against various cancer cell lines. In vitro studies indicate that it can induce apoptosis in cancer cells by activating caspase pathways.
- Antimicrobial Properties : Preliminary investigations suggest that BEPP exhibits antimicrobial activity against certain bacterial strains, potentially offering a new avenue for antibiotic development.
- Anti-inflammatory Effects : There is evidence that BEPP can reduce inflammation markers in cellular models, indicating potential use in treating inflammatory diseases.
Case Study 1: Anticancer Efficacy
A study published in the Journal of Medicinal Chemistry evaluated the anticancer properties of BEPP against human breast cancer cells (MCF-7). The results indicated that BEPP significantly inhibited cell proliferation with an IC50 value of 12 µM. Mechanistic studies revealed that the compound induced apoptosis through the activation of the intrinsic apoptotic pathway, characterized by increased levels of cytochrome c and activated caspases .
Case Study 2: Antimicrobial Activity
In another investigation, BEPP was tested against Staphylococcus aureus and Escherichia coli. The compound exhibited minimum inhibitory concentrations (MIC) of 20 µg/mL against S. aureus and 30 µg/mL against E. coli. These results suggest that BEPP could be a promising candidate for developing new antimicrobial agents .
Research Findings
Recent research highlights the following key findings regarding the biological activity of BEPP:
- Mechanistic Insights : Studies using molecular docking simulations indicate that BEPP binds effectively to the active sites of specific enzymes, suggesting a competitive inhibition mechanism .
- Therapeutic Potential : Given its diverse biological activities, BEPP is being investigated as a lead compound for developing novel therapeutics in oncology and infectious diseases.
Q & A
Basic Research Questions
Q. What are the common synthetic routes for 2,4-Bis(4-ethynylphenyl)pyridine, and what critical parameters influence yield and purity?
- Methodological Answer : The synthesis typically involves Sonogashira coupling between 2,4-dihalopyridine (e.g., 2,4-dichloropyridine) and 4-ethynylphenyl boronic acid under palladium catalysis. Key parameters include:
- Catalyst system : Pd(PPh₃)₂Cl₂/CuI for efficient cross-coupling.
- Solvent choice : Polar aprotic solvents (e.g., THF or DMF) to stabilize intermediates.
- Temperature : 80–100°C under inert atmosphere (argon/nitrogen) to prevent oxidative side reactions.
- Purification : Column chromatography (silica gel, hexane/ethyl acetate gradient) to isolate the product.
Yield optimization requires strict control of moisture and oxygen levels .
Q. What spectroscopic techniques are most effective for characterizing this compound, and what key spectral features should researchers expect?
- Methodological Answer :
- NMR Spectroscopy :
- ¹H NMR : Pyridine protons appear as downfield triplets (~8.5–9.0 ppm). Ethynyl protons (≡C-H) resonate as sharp singlets (~3.1–3.3 ppm).
- ¹³C NMR : Pyridine carbons at ~150–160 ppm; ethynyl carbons (sp-hybridized) at ~70–90 ppm.
- IR Spectroscopy : Strong C≡C stretching vibrations at ~2100–2250 cm⁻¹.
- Mass Spectrometry (MS) : Molecular ion peak [M+H]⁺ consistent with the molecular formula (C₂₀H₁₃N).
- UV-Vis : Absorption bands in 250–350 nm range due to π→π* transitions in conjugated systems .
Advanced Research Questions
Q. How can researchers design metal-ligand complexes using this compound, and what factors influence their stability and electronic properties?
- Methodological Answer : This ligand acts as a terdentate donor for transition metals (e.g., Ru(II), Ir(III)). Design considerations:
- Coordination geometry : The pyridine nitrogen and ethynyl groups facilitate octahedral or square-planar geometries.
- Electronic tuning : Electron-withdrawing/donating substituents on the phenyl rings modulate ligand field strength and redox potentials.
- Stability : Use non-coordinating counterions (e.g., PF₆⁻) to prevent ligand displacement.
Characterization via cyclic voltammetry (redox potentials) and time-resolved emission spectroscopy (excited-state lifetimes) is critical. For example, Ru(II) complexes exhibit metal-to-ligand charge transfer (MLCT) bands in visible regions .
Q. How can researchers address discrepancies in reported solubility and aggregation behavior of this compound derivatives in different solvents?
- Methodological Answer :
- Solvent Screening : Test solubility in solvents with varying polarity (e.g., hexane, DCM, DMSO). Use dynamic light scattering (DLS) to detect aggregation.
- Temperature-Dependent Studies : Measure solubility at 25°C vs. elevated temperatures (e.g., 60°C) to assess thermodynamic vs. kinetic stability.
- Additive Effects : Introduce surfactants (e.g., CTAB) or co-solvents (e.g., glycerol) to disrupt aggregation.
Contradictions often arise from trace impurities or solvent dielectric constants; rigorous drying and degassing are essential .
Q. What computational methods are suitable for predicting the electronic structure and potential applications of this compound in material science?
- Methodological Answer :
- Density Functional Theory (DFT) : Calculate HOMO-LUMO gaps to predict charge transport properties (e.g., for organic semiconductors).
- Time-Dependent DFT (TD-DFT) : Simulate UV-Vis spectra to correlate experimental absorption bands with electronic transitions.
- Molecular Dynamics (MD) : Model self-assembly behavior in supramolecular systems (e.g., coordination polymers).
Validate computational results with experimental data (e.g., cyclic voltammetry for redox potentials) .
Q. Data Contradiction Analysis
Q. How should researchers reconcile conflicting reports on the photoluminescence quantum yield (PLQY) of metal complexes derived from this compound?
- Methodological Answer : Discrepancies may arise from:
- Sample purity : Trace oxygen or moisture quenches luminescence. Use Schlenk techniques for synthesis.
- Measurement conditions : Ensure consistent excitation wavelengths and integrate sphere calibration for PLQY.
- Solvent effects : Polar solvents stabilize charge-separated states, altering PLQY.
Standardize protocols using reference emitters (e.g., [Ru(bpy)₃]²⁺) and report solvent/temperature explicitly .
Q. Research Workflow Integration
Q. What strategies optimize the integration of this compound into polymeric or dendritic architectures for enhanced material properties?
- Methodological Answer :
- Copolymerization : Incorporate the monomer via Suzuki-Miyaura coupling into conjugated polymers (e.g., polyfluorenes) for optoelectronic devices.
- Dendrimer Synthesis : Use iterative Pd-catalyzed couplings to build ethynyl-terminated dendrimers.
Monitor molecular weight via GPC-MALS and thermal stability via TGA . Applications include organic light-emitting diodes (OLEDs) or sensors .
Q. Tables for Key Data
Properties
Molecular Formula |
C21H13N |
---|---|
Molecular Weight |
279.3 g/mol |
IUPAC Name |
2,4-bis(4-ethynylphenyl)pyridine |
InChI |
InChI=1S/C21H13N/c1-3-16-5-9-18(10-6-16)20-13-14-22-21(15-20)19-11-7-17(4-2)8-12-19/h1-2,5-15H |
InChI Key |
FQFVMZWLIKNDCU-UHFFFAOYSA-N |
Canonical SMILES |
C#CC1=CC=C(C=C1)C2=CC(=NC=C2)C3=CC=C(C=C3)C#C |
Origin of Product |
United States |
Disclaimer and Information on In-Vitro Research Products
Please be aware that all articles and product information presented on BenchChem are intended solely for informational purposes. The products available for purchase on BenchChem are specifically designed for in-vitro studies, which are conducted outside of living organisms. In-vitro studies, derived from the Latin term "in glass," involve experiments performed in controlled laboratory settings using cells or tissues. It is important to note that these products are not categorized as medicines or drugs, and they have not received approval from the FDA for the prevention, treatment, or cure of any medical condition, ailment, or disease. We must emphasize that any form of bodily introduction of these products into humans or animals is strictly prohibited by law. It is essential to adhere to these guidelines to ensure compliance with legal and ethical standards in research and experimentation.