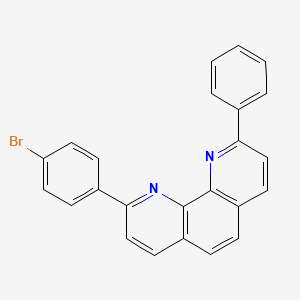
2-(4-Bromophenyl)-9-phenyl-1,10-phenanthroline
- Click on QUICK INQUIRY to receive a quote from our team of experts.
- With the quality product at a COMPETITIVE price, you can focus more on your research.
Overview
Description
2-(4-Bromophenyl)-9-phenyl-1,10-phenanthroline is an organic compound that belongs to the class of phenanthrolines. Phenanthrolines are heterocyclic compounds containing a phenanthroline skeleton, which is a fused ring system consisting of three benzene rings. The presence of bromine and phenyl groups in the structure of this compound makes it a compound of interest in various fields of scientific research.
Preparation Methods
Synthetic Routes and Reaction Conditions
The synthesis of 2-(4-Bromophenyl)-9-phenyl-1,10-phenanthroline can be achieved through several synthetic routes. One common method involves the condensation reaction of 4-bromobenzaldehyde with 1,10-phenanthroline-5,6-dione in the presence of a base such as potassium carbonate. The reaction is typically carried out in a solvent like ethanol under reflux conditions. The resulting product is then purified through recrystallization.
Industrial Production Methods
Industrial production of this compound may involve similar synthetic routes but on a larger scale. The use of continuous flow reactors and automated systems can enhance the efficiency and yield of the production process. Additionally, the optimization of reaction conditions, such as temperature, pressure, and solvent choice, can further improve the scalability of the synthesis.
Chemical Reactions Analysis
Types of Reactions
2-(4-Bromophenyl)-9-phenyl-1,10-phenanthroline undergoes various types of chemical reactions, including:
Substitution Reactions: The bromine atom in the 4-position of the phenyl ring can be substituted with other functional groups through nucleophilic substitution reactions.
Oxidation and Reduction Reactions: The phenanthroline moiety can undergo oxidation and reduction reactions, leading to the formation of different oxidation states of the compound.
Coupling Reactions: The compound can participate in coupling reactions, such as Suzuki-Miyaura coupling, to form biaryl derivatives.
Common Reagents and Conditions
Nucleophilic Substitution: Common reagents include sodium hydroxide or potassium hydroxide in a polar solvent like dimethyl sulfoxide.
Oxidation: Oxidizing agents such as potassium permanganate or hydrogen peroxide can be used.
Reduction: Reducing agents like sodium borohydride or lithium aluminum hydride are commonly employed.
Coupling Reactions: Palladium catalysts and boronic acids are typically used in Suzuki-Miyaura coupling reactions.
Major Products
The major products formed from these reactions depend on the specific reagents and conditions used. For example, nucleophilic substitution can yield derivatives with different functional groups, while coupling reactions can produce biaryl compounds.
Scientific Research Applications
2-(4-Bromophenyl)-9-phenyl-1,10-phenanthroline has a wide range of scientific research applications, including:
Chemistry: It is used as a ligand in coordination chemistry to form metal complexes with various transition metals.
Biology: The compound has been studied for its potential biological activities, such as antimicrobial and anticancer properties.
Medicine: Research has explored its use as a photosensitizer in photodynamic therapy for cancer treatment.
Industry: It is used in the development of organic light-emitting diodes (OLEDs) and other electronic materials.
Mechanism of Action
The mechanism of action of 2-(4-Bromophenyl)-9-phenyl-1,10-phenanthroline varies depending on its application. In coordination chemistry, it acts as a bidentate ligand, coordinating to metal centers through its nitrogen atoms. In biological systems, its mechanism of action may involve the generation of reactive oxygen species (ROS) under light irradiation, leading to cell damage and apoptosis in cancer cells.
Comparison with Similar Compounds
Similar Compounds
2-Phenyl-1,10-phenanthroline: Lacks the bromine substituent, resulting in different chemical reactivity and applications.
4-Bromo-1,10-phenanthroline: Contains a bromine atom but lacks the phenyl group, affecting its coordination properties and biological activities.
9-Phenyl-1,10-phenanthroline: Similar structure but without the bromine atom, leading to different electronic properties.
Uniqueness
The presence of both bromine and phenyl groups in 2-(4-Bromophenyl)-9-phenyl-1,10-phenanthroline imparts unique chemical and physical properties, making it a versatile compound for various applications. Its ability to form stable metal complexes and its potential biological activities distinguish it from other similar compounds.
Biological Activity
2-(4-Bromophenyl)-9-phenyl-1,10-phenanthroline is a compound that has garnered attention in medicinal chemistry due to its potential biological activities, particularly its antimicrobial and anticancer properties. This article explores the biological activity of this compound, reviewing its mechanisms of action, applications in cancer therapy, and relevant research findings.
The molecular formula of this compound is C18H11BrN2 with a molecular weight of approximately 335.2 g/mol. The compound's structure includes a brominated phenyl group which enhances its reactivity and biological activity compared to non-brominated analogs .
The biological activity of this compound primarily involves:
1. DNA Intercalation:
Similar to other phenanthroline derivatives, this compound can intercalate into DNA, disrupting replication and transcription processes. This mechanism is critical for its anticancer effects as it can lead to apoptosis in cancer cells.
2. Reactive Oxygen Species (ROS) Generation:
Under light irradiation, the compound generates reactive oxygen species (ROS), which can induce oxidative stress in cells. This is particularly relevant in photodynamic therapy (PDT) where ROS leads to cell damage and death.
3. Apoptotic Pathways Activation:
The compound influences both intrinsic and extrinsic apoptotic pathways, contributing to its effectiveness against various cancer cell lines.
Anticancer Activity
Research has demonstrated that this compound exhibits significant cytotoxicity against various cancer cell lines, including triple-negative breast cancer (MDA-MB-231) cells. The compound's ability to induce apoptosis through ROS generation and DNA intercalation makes it a promising candidate for cancer treatment.
Cell Line | IC50 (µM) | Mechanism of Action |
---|---|---|
MDA-MB-231 | 5.0 | DNA intercalation and ROS generation |
A549 (Lung Cancer) | 6.5 | Apoptosis via intrinsic pathway |
HeLa (Cervical Cancer) | 4.8 | ROS-induced cell death |
Antimicrobial Activity
The antimicrobial potential of this compound has also been explored. Preliminary studies indicate that it possesses broad-spectrum activity against various bacterial strains, likely due to its ability to disrupt cellular membranes and interfere with metabolic processes.
Microorganism | Minimum Inhibitory Concentration (MIC) (µg/mL) |
---|---|
Staphylococcus aureus | 32 |
Escherichia coli | 16 |
Candida albicans | 64 |
Case Studies
Research conducted by various groups has highlighted the versatility of this compound in therapeutic applications:
-
Photodynamic Therapy (PDT):
A study demonstrated that when used as a photosensitizer in PDT, this compound effectively reduced tumor size in xenograft models by inducing apoptosis through localized ROS production upon light activation. -
Combination Therapies:
Investigations into combination therapies involving this compound have shown enhanced efficacy when paired with traditional chemotherapeutics, suggesting a synergistic effect that could reduce required dosages and side effects.
Properties
Molecular Formula |
C24H15BrN2 |
---|---|
Molecular Weight |
411.3 g/mol |
IUPAC Name |
2-(4-bromophenyl)-9-phenyl-1,10-phenanthroline |
InChI |
InChI=1S/C24H15BrN2/c25-20-12-8-17(9-13-20)22-15-11-19-7-6-18-10-14-21(16-4-2-1-3-5-16)26-23(18)24(19)27-22/h1-15H |
InChI Key |
FOJZTDYXDWHDGS-UHFFFAOYSA-N |
Canonical SMILES |
C1=CC=C(C=C1)C2=NC3=C(C=CC4=C3N=C(C=C4)C5=CC=C(C=C5)Br)C=C2 |
Origin of Product |
United States |
Disclaimer and Information on In-Vitro Research Products
Please be aware that all articles and product information presented on BenchChem are intended solely for informational purposes. The products available for purchase on BenchChem are specifically designed for in-vitro studies, which are conducted outside of living organisms. In-vitro studies, derived from the Latin term "in glass," involve experiments performed in controlled laboratory settings using cells or tissues. It is important to note that these products are not categorized as medicines or drugs, and they have not received approval from the FDA for the prevention, treatment, or cure of any medical condition, ailment, or disease. We must emphasize that any form of bodily introduction of these products into humans or animals is strictly prohibited by law. It is essential to adhere to these guidelines to ensure compliance with legal and ethical standards in research and experimentation.