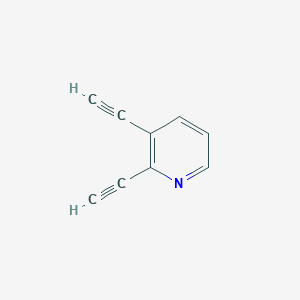
2,3-Diethynylpyridine
- Click on QUICK INQUIRY to receive a quote from our team of experts.
- With the quality product at a COMPETITIVE price, you can focus more on your research.
Overview
Description
2,3-Diethynylpyridine is a chemical compound characterized by the presence of two ethynyl groups attached to the 2nd and 3rd positions of a pyridine ring. This compound is of significant interest in organic chemistry due to its unique structural features and reactivity, making it a valuable building block for various chemical syntheses and applications.
Preparation Methods
Synthetic Routes and Reaction Conditions: 2,3-Diethynylpyridine can be synthesized through various methods. One common approach involves the reaction of 2,3-dibromopyridine with terminal alkynes under Sonogashira coupling conditions. This reaction typically requires a palladium catalyst, a copper co-catalyst, and a base such as triethylamine, conducted in an inert atmosphere .
Industrial Production Methods: While specific industrial production methods for this compound are not extensively documented, the general principles of large-scale organic synthesis apply. These include optimizing reaction conditions for yield and purity, scaling up the reaction while maintaining safety and efficiency, and employing continuous flow techniques to enhance production rates.
Chemical Reactions Analysis
Types of Reactions: 2,3-Diethynylpyridine undergoes various chemical reactions, including:
Oxidation: The ethynyl groups can be oxidized to form diketones or carboxylic acids.
Reduction: Reduction of the ethynyl groups can yield alkenes or alkanes.
Substitution: The pyridine ring can undergo electrophilic and nucleophilic substitution reactions.
Common Reagents and Conditions:
Oxidation: Reagents such as potassium permanganate or osmium tetroxide.
Reduction: Catalysts like palladium on carbon (Pd/C) or hydrogen gas.
Substitution: Halogenating agents, nucleophiles like amines or thiols.
Major Products:
Oxidation: Formation of diketones or carboxylic acids.
Reduction: Formation of alkenes or alkanes.
Substitution: Various substituted pyridines depending on the nucleophile used
Scientific Research Applications
2,3-Diethynylpyridine has a wide range of applications in scientific research:
Chemistry: Used as a building block for synthesizing complex organic molecules and polymers.
Biology: Investigated for its potential as a ligand in coordination chemistry and its interactions with biological macromolecules.
Medicine: Explored for its potential in drug development, particularly in designing molecules with specific biological activities.
Industry: Utilized in the production of advanced materials, including conductive polymers and nanomaterials .
Mechanism of Action
The mechanism of action of 2,3-diethynylpyridine largely depends on its application. In coordination chemistry, it acts as a ligand, forming complexes with metal ions. The ethynyl groups can participate in π-π interactions and conjugation, influencing the electronic properties of the resulting complexes. In biological systems, it may interact with enzymes or receptors, modulating their activity through binding interactions .
Comparison with Similar Compounds
2,5-Diethynylpyridine: Similar structure but with ethynyl groups at the 2nd and 5th positions.
2,6-Diethynylpyridine: Ethynyl groups at the 2nd and 6th positions, often used in coordination chemistry.
3,4-Diethynylpyridine: Ethynyl groups at the 3rd and 4th positions, differing in reactivity and applications.
Uniqueness: 2,3-Diethynylpyridine is unique due to the specific positioning of its ethynyl groups, which imparts distinct electronic and steric properties. This makes it particularly useful in synthesizing specific molecular frameworks and studying unique chemical interactions.
Biological Activity
2,3-Diethynylpyridine is a compound that has garnered attention in various fields of research, particularly in medicinal chemistry and materials science. This article reviews the biological activity of this compound, focusing on its potential therapeutic applications, mechanisms of action, and interactions with biological systems.
This compound is characterized by two ethynyl groups attached to the pyridine ring. This structure contributes to its unique chemical reactivity and ability to form coordination complexes. The compound is synthesized through various methods, including Sonogashira coupling reactions, which allow for the introduction of ethynyl groups onto the pyridine framework .
Anticancer Activity
Research has indicated that compounds related to diethynylpyridine exhibit significant anticancer properties. For instance, studies have shown that certain derivatives can bind to cisplatin, a well-known anticancer drug, enhancing its efficacy . The binding interactions were monitored using NMR spectroscopy, revealing that this compound derivatives could stabilize cisplatin in solution, potentially leading to improved therapeutic outcomes.
Enzyme Inhibition
Another area of interest is the inhibition of enzymes involved in critical biological pathways. For example, some studies have explored the ability of diethynylpyridine derivatives to inhibit cytochrome P450 enzymes, which play a significant role in drug metabolism . Inhibition of these enzymes can lead to altered pharmacokinetics for various drugs, impacting their effectiveness and safety profiles.
Binding Studies
Binding affinity studies using NMR titration have demonstrated that this compound can interact with various biological molecules. For instance, it has been shown to form stable complexes with anions through hydrogen bonding and π-π stacking interactions . These interactions are crucial for understanding how this compound can modulate biological processes at the molecular level.
Case Studies and Research Findings
Several case studies highlight the potential applications of this compound:
- Anticancer Drug Development : A study investigated the use of this compound as a scaffold for developing novel anticancer agents. The derivatives showed promising activity against various cancer cell lines and were further optimized for improved selectivity and potency.
- Antiviral Properties : Research has also explored the antiviral properties of diethynylpyridine derivatives against viruses such as Hepatitis C. The compounds demonstrated inhibitory activity on viral proteases, suggesting a potential role in antiviral therapy .
- Material Science Applications : Beyond pharmacological applications, this compound has been utilized in creating functional materials due to its ability to form coordination complexes with metals. These materials have applications in sensors and catalysis.
Data Summary
The following table summarizes key findings related to the biological activity of this compound:
Properties
Molecular Formula |
C9H5N |
---|---|
Molecular Weight |
127.14 g/mol |
IUPAC Name |
2,3-diethynylpyridine |
InChI |
InChI=1S/C9H5N/c1-3-8-6-5-7-10-9(8)4-2/h1-2,5-7H |
InChI Key |
MICZGSDPOZUBDZ-UHFFFAOYSA-N |
Canonical SMILES |
C#CC1=C(N=CC=C1)C#C |
Origin of Product |
United States |
Disclaimer and Information on In-Vitro Research Products
Please be aware that all articles and product information presented on BenchChem are intended solely for informational purposes. The products available for purchase on BenchChem are specifically designed for in-vitro studies, which are conducted outside of living organisms. In-vitro studies, derived from the Latin term "in glass," involve experiments performed in controlled laboratory settings using cells or tissues. It is important to note that these products are not categorized as medicines or drugs, and they have not received approval from the FDA for the prevention, treatment, or cure of any medical condition, ailment, or disease. We must emphasize that any form of bodily introduction of these products into humans or animals is strictly prohibited by law. It is essential to adhere to these guidelines to ensure compliance with legal and ethical standards in research and experimentation.