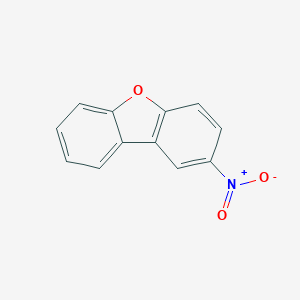
2-Nitrodibenzofuran
Overview
Description
2-Nitrodibenzofuran is an organic compound belonging to the class of nitroaromatic compounds It is characterized by a dibenzofuran core structure with a nitro group (-NO2) attached to the second position
Mechanism of Action
Target of Action
The primary target of 2-Nitrodibenzofuran (NDBF) is the thiol group in peptides . NDBF acts as a photoremovable protecting group for the thiol group, which is crucial in peptide chemistry .
Mode of Action
NDBF interacts with its target by masking the thiol group in cysteine-containing peptides . This is achieved by synthesizing Fmoc-Cys(NDBF)-OH and incorporating that residue into peptides by standard solid-phase peptide synthesis procedures . Upon irradiation with 365 nm light or two-photon excitation with 800 nm light, efficient deprotection occurs .
Biochemical Pathways
The biochemical pathway affected by NDBF involves the uncaging of the thiol group in peptides . This uncaging process is carried out using a peptide derived from the protein K-Ras4B, a known substrate for protein farnesyltransferase . Upon irradiation of the NDBF-caged peptide in the presence of the enzyme, the formation of the farnesylated product occurs .
Pharmacokinetics
The pharmacokinetics of NDBF involve its efficient deprotection upon irradiation . The high cleavage efficiency devoid of side reactions and significant two-photon cross-section of NDBF render it superior to Bhc for thiol group caging . .
Result of Action
The molecular and cellular effects of NDBF’s action involve the migration of the peptide from the cytosol/Golgi to the plasma membrane due to enzymatic palmitoylation . This occurs when human ovarian carcinoma (SKOV3) cells are incubated with an NDBF-caged version of a farnesylated peptide followed by UV irradiation .
Action Environment
The action of NDBF is influenced by light, specifically 365 nm light or two-photon excitation with 800 nm light . The ability of light to traverse various chemical and biological barriers and be modulated by time and amplitude makes light-regulated molecules like NDBF unique tools for a plethora of applications in the areas of chemistry and biology .
Biochemical Analysis
Biochemical Properties
2-Nitrodibenzofuran has been used as a photoremovable protecting group in peptide chemistry . It interacts with cysteine residues in peptides, masking the thiol group . Upon irradiation with light, the this compound group is removed, revealing the thiol group . This interaction is crucial in the study of proteins and enzymes that contain cysteine residues .
Cellular Effects
In cellular studies, this compound has been used to control the activity of peptides within cells . For example, a peptide derived from the protein K-Ras4B was caged with this compound . Upon irradiation, the peptide was activated, leading to changes in cell signaling pathways and gene expression . This demonstrates the potential of this compound to influence cellular function.
Molecular Mechanism
The mechanism of action of this compound involves its ability to absorb light and undergo a photochemical reaction . When irradiated, this compound undergoes a structural change that leads to its removal from the peptide . This uncaging process allows the peptide to interact with other biomolecules, potentially leading to changes in enzyme activity and gene expression .
Temporal Effects in Laboratory Settings
The effects of this compound can be controlled temporally by adjusting the timing and duration of light exposure This allows researchers to study the effects of the compound over time
Metabolic Pathways
It is known that dibenzofurans can be degraded by bacteria through lateral and angular dioxygenation pathways
Transport and Distribution
It is known that the compound can penetrate cells and interact with intracellular peptides
Subcellular Localization
It has been shown that peptides caged with this compound can migrate from the cytosol/Golgi to the plasma membrane upon irradiation This suggests that this compound may influence the subcellular localization of peptides
Preparation Methods
Synthetic Routes and Reaction Conditions
The synthesis of 2-Nitrodibenzofuran can be achieved through several methods:
Electrophilic Nitration: This involves the nitration of dibenzofuran using a mixture of nitric acid and acetic acid.
Ipso Substitution: This method involves the substitution of a halogen atom in dibenzofuran with a nitro group using tetranitromethane as the nitrating agent.
Condensation Reactions: Condensation of salicylic aldehydes with bromonitromethane followed by cyclization can also yield this compound.
Industrial Production Methods
Industrial production of this compound typically involves large-scale nitration processes using optimized reaction conditions to maximize yield and purity. The choice of nitrating agents and reaction conditions is crucial to ensure the selective formation of the desired nitro isomer.
Chemical Reactions Analysis
2-Nitrodibenzofuran undergoes various chemical reactions, including:
Substitution: The nitro group can participate in nucleophilic aromatic substitution reactions, where it is replaced by other nucleophiles.
Common reagents used in these reactions include hydrogen gas, metal hydrides, and various nucleophiles. The major products formed depend on the specific reaction conditions and reagents used.
Scientific Research Applications
2-Nitrodibenzofuran has several scientific research applications:
Peptide Chemistry: It is used as a photoremovable protecting group for thiol groups in peptides.
Biological Studies: The compound is used in the development of light-sensitive biomaterials and in studies involving light-regulated biological processes.
Material Science: Its unique photochemical properties make it useful in the development of advanced materials with light-responsive behaviors.
Comparison with Similar Compounds
2-Nitrodibenzofuran can be compared with other nitroaromatic compounds such as:
2-Nitrobenzofuran: Similar in structure but with different reactivity and applications.
Brominated Hydroxycoumarin: Used as a protecting group but less efficient compared to this compound.
2-Nitronaphtho[2,1-b]furan: Known for its strong mutagenic properties but differs in its biological applications.
The uniqueness of this compound lies in its superior efficiency as a photoremovable protecting group and its broad range of applications in scientific research.
Properties
IUPAC Name |
2-nitrodibenzofuran | |
---|---|---|
Source | PubChem | |
URL | https://pubchem.ncbi.nlm.nih.gov | |
Description | Data deposited in or computed by PubChem | |
InChI |
InChI=1S/C12H7NO3/c14-13(15)8-5-6-12-10(7-8)9-3-1-2-4-11(9)16-12/h1-7H | |
Source | PubChem | |
URL | https://pubchem.ncbi.nlm.nih.gov | |
Description | Data deposited in or computed by PubChem | |
InChI Key |
YPFPLEUXZNAAEE-UHFFFAOYSA-N | |
Source | PubChem | |
URL | https://pubchem.ncbi.nlm.nih.gov | |
Description | Data deposited in or computed by PubChem | |
Canonical SMILES |
C1=CC=C2C(=C1)C3=C(O2)C=CC(=C3)[N+](=O)[O-] | |
Source | PubChem | |
URL | https://pubchem.ncbi.nlm.nih.gov | |
Description | Data deposited in or computed by PubChem | |
Molecular Formula |
C12H7NO3 | |
Source | PubChem | |
URL | https://pubchem.ncbi.nlm.nih.gov | |
Description | Data deposited in or computed by PubChem | |
DSSTOX Substance ID |
DTXSID60175086 | |
Record name | Dibenzofuran, 2-nitro- | |
Source | EPA DSSTox | |
URL | https://comptox.epa.gov/dashboard/DTXSID60175086 | |
Description | DSSTox provides a high quality public chemistry resource for supporting improved predictive toxicology. | |
Molecular Weight |
213.19 g/mol | |
Source | PubChem | |
URL | https://pubchem.ncbi.nlm.nih.gov | |
Description | Data deposited in or computed by PubChem | |
CAS No. |
20927-95-1 | |
Record name | 2-Nitrodibenzofuran | |
Source | CAS Common Chemistry | |
URL | https://commonchemistry.cas.org/detail?cas_rn=20927-95-1 | |
Description | CAS Common Chemistry is an open community resource for accessing chemical information. Nearly 500,000 chemical substances from CAS REGISTRY cover areas of community interest, including common and frequently regulated chemicals, and those relevant to high school and undergraduate chemistry classes. This chemical information, curated by our expert scientists, is provided in alignment with our mission as a division of the American Chemical Society. | |
Explanation | The data from CAS Common Chemistry is provided under a CC-BY-NC 4.0 license, unless otherwise stated. | |
Record name | Dibenzofuran, 2-nitro- | |
Source | ChemIDplus | |
URL | https://pubchem.ncbi.nlm.nih.gov/substance/?source=chemidplus&sourceid=0020927951 | |
Description | ChemIDplus is a free, web search system that provides access to the structure and nomenclature authority files used for the identification of chemical substances cited in National Library of Medicine (NLM) databases, including the TOXNET system. | |
Record name | Dibenzofuran, 2-nitro- | |
Source | EPA DSSTox | |
URL | https://comptox.epa.gov/dashboard/DTXSID60175086 | |
Description | DSSTox provides a high quality public chemistry resource for supporting improved predictive toxicology. | |
Synthesis routes and methods
Procedure details
Retrosynthesis Analysis
AI-Powered Synthesis Planning: Our tool employs the Template_relevance Pistachio, Template_relevance Bkms_metabolic, Template_relevance Pistachio_ringbreaker, Template_relevance Reaxys, Template_relevance Reaxys_biocatalysis model, leveraging a vast database of chemical reactions to predict feasible synthetic routes.
One-Step Synthesis Focus: Specifically designed for one-step synthesis, it provides concise and direct routes for your target compounds, streamlining the synthesis process.
Accurate Predictions: Utilizing the extensive PISTACHIO, BKMS_METABOLIC, PISTACHIO_RINGBREAKER, REAXYS, REAXYS_BIOCATALYSIS database, our tool offers high-accuracy predictions, reflecting the latest in chemical research and data.
Strategy Settings
Precursor scoring | Relevance Heuristic |
---|---|
Min. plausibility | 0.01 |
Model | Template_relevance |
Template Set | Pistachio/Bkms_metabolic/Pistachio_ringbreaker/Reaxys/Reaxys_biocatalysis |
Top-N result to add to graph | 6 |
Feasible Synthetic Routes
Q1: What are the different methods for synthesizing 2-Nitrodibenzofuran and how do they differ in regioselectivity?
A1: Two main synthetic routes yield this compound with differing regioselectivity:
Q2: Can this compound be synthesized through photochemical reactions, and what are the potential applications of this approach?
A2: While not directly producing this compound, research demonstrates the feasibility of using photochemical reactions to create dibenzofuran derivatives []. For instance, UV irradiation of 3,5-diiodo-4-(4'-methyoxphenoxy)nitrobenzene yields 4-iodo-8-methoxy-2-nitrodibenzofuran []. This method shows promise for developing novel, rigid thyroid hormone analogs by utilizing the functionalized dibenzofuran framework.
Q3: Has this compound been used in the development of photocleavable protecting groups, and what mechanistic insights are available?
A3: Research indicates the use of a this compound derivative, specifically 3-methyl-2-nitrodibenzofuran (NDBF), in synthesizing FerriCast (FC-NDBF), a potential photocleavable protecting group for Fe(3+) []. Studying the photochemistry of FC-NDBF and similar compounds provides valuable insights into the uncaging quantum yields of ligands derived from nitrobenzhydrol. This highlights the potential of this compound derivatives in photochemical applications.
Disclaimer and Information on In-Vitro Research Products
Please be aware that all articles and product information presented on BenchChem are intended solely for informational purposes. The products available for purchase on BenchChem are specifically designed for in-vitro studies, which are conducted outside of living organisms. In-vitro studies, derived from the Latin term "in glass," involve experiments performed in controlled laboratory settings using cells or tissues. It is important to note that these products are not categorized as medicines or drugs, and they have not received approval from the FDA for the prevention, treatment, or cure of any medical condition, ailment, or disease. We must emphasize that any form of bodily introduction of these products into humans or animals is strictly prohibited by law. It is essential to adhere to these guidelines to ensure compliance with legal and ethical standards in research and experimentation.