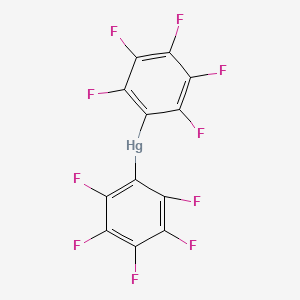
Bis(pentafluorophenyl)mercury
- Click on QUICK INQUIRY to receive a quote from our team of experts.
- With the quality product at a COMPETITIVE price, you can focus more on your research.
Overview
Description
Bis(pentafluorophenyl)mercury is an organomercury compound with the chemical formula Hg(C₆F₅)₂ It is characterized by the presence of two pentafluorophenyl groups attached to a central mercury atom
Preparation Methods
Synthetic Routes and Reaction Conditions
Bis(pentafluorophenyl)mercury can be synthesized through the reaction of pentafluorophenylmagnesium bromide with mercuric chloride. The reaction is typically carried out in an inert atmosphere to prevent oxidation and involves the following steps:
- Preparation of pentafluorophenylmagnesium bromide by reacting pentafluorobenzene with magnesium in the presence of a suitable solvent such as diethyl ether.
- Addition of mercuric chloride to the reaction mixture, resulting in the formation of this compound and magnesium bromide as a byproduct.
The reaction can be represented as:
2C6F5MgBr+HgCl2→Hg(C6F5)2+2MgBrCl
Industrial Production Methods
While the industrial production methods for this compound are not extensively documented, the synthesis typically follows similar principles as laboratory-scale preparation, with considerations for scaling up the reaction and ensuring safety protocols are in place due to the toxicity of mercury compounds.
Chemical Reactions Analysis
Types of Reactions
Bis(pentafluorophenyl)mercury undergoes various chemical reactions, including:
Substitution Reactions: The pentafluorophenyl groups can be substituted by other nucleophiles, leading to the formation of new organomercury compounds.
Coordination Reactions: This compound can form coordination complexes with ligands such as phosphane oxides, resulting in the formation of crystalline σ-donor complexes.
Common Reagents and Conditions
Nucleophiles: Common nucleophiles used in substitution reactions include halides, alkoxides, and thiolates.
Solvents: Reactions are typically carried out in solvents such as acetonitrile or diethyl ether to ensure solubility and stability of the reactants and products.
Major Products
The major products formed from these reactions depend on the specific nucleophiles and ligands used. For example, the reaction with bis(diphenylphosphano)methane P,P’-dioxide results in the formation of a crystalline σ-donor complex .
Scientific Research Applications
Bis(pentafluorophenyl)mercury has several applications in scientific research, including:
Chemistry: It is used as a precursor for the synthesis of other organomercury compounds and coordination complexes.
Biology and Medicine: While direct applications in biology and medicine are limited due to the toxicity of mercury compounds, this compound can be used in studies related to the interaction of mercury with biological molecules.
Industry: The compound’s unique properties make it useful in materials science and catalysis research.
Mechanism of Action
The mechanism by which bis(pentafluorophenyl)mercury exerts its effects involves the coordination of the mercury atom with various ligands. The pentafluorophenyl groups enhance the stability of the compound and influence its reactivity by reducing the Lewis acidity of the mercury center . This allows for the formation of stable coordination complexes with a variety of ligands, which can be studied for their structural and electronic properties.
Comparison with Similar Compounds
Similar Compounds
Diphenylmercury (Hg(C₆H₅)₂): Unlike bis(pentafluorophenyl)mercury, diphenylmercury lacks the electron-withdrawing fluorine atoms, resulting in different reactivity and stability.
Methylpentafluorophenylmercury (Hg(C₆F₅)(CH₃)):
Uniqueness
This compound is unique due to the presence of two pentafluorophenyl groups, which significantly influence its chemical behavior. The electron-withdrawing effect of the fluorine atoms enhances the stability of the compound and allows for the formation of unique coordination complexes that are not easily achievable with other organomercury compounds .
Properties
CAS No. |
973-17-1 |
---|---|
Molecular Formula |
C12F10Hg |
Molecular Weight |
534.70 g/mol |
IUPAC Name |
bis(2,3,4,5,6-pentafluorophenyl)mercury |
InChI |
InChI=1S/2C6F5.Hg/c2*7-2-1-3(8)5(10)6(11)4(2)9; |
InChI Key |
UAYZCFIZMZPZOZ-UHFFFAOYSA-N |
Canonical SMILES |
C1(=C(C(=C(C(=C1F)F)[Hg]C2=C(C(=C(C(=C2F)F)F)F)F)F)F)F |
Origin of Product |
United States |
Disclaimer and Information on In-Vitro Research Products
Please be aware that all articles and product information presented on BenchChem are intended solely for informational purposes. The products available for purchase on BenchChem are specifically designed for in-vitro studies, which are conducted outside of living organisms. In-vitro studies, derived from the Latin term "in glass," involve experiments performed in controlled laboratory settings using cells or tissues. It is important to note that these products are not categorized as medicines or drugs, and they have not received approval from the FDA for the prevention, treatment, or cure of any medical condition, ailment, or disease. We must emphasize that any form of bodily introduction of these products into humans or animals is strictly prohibited by law. It is essential to adhere to these guidelines to ensure compliance with legal and ethical standards in research and experimentation.