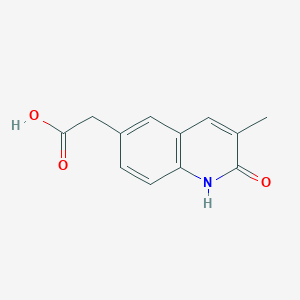
2-(3-Methyl-2-oxo-1,2-dihydroquinolin-6-yl)acetic acid
Overview
Description
2-(3-Methyl-2-oxo-1,2-dihydroquinolin-6-yl)acetic acid is a quinoline derivative characterized by a 1,2-dihydroquinolin-2-one core substituted with a methyl group at position 3 and an acetic acid moiety at position 6. Its molecular formula is C₁₂H₁₁NO₃ (calculated molecular weight: 217.22 g/mol). The acetic acid group enhances solubility and reactivity, facilitating its use in medicinal chemistry .
Scientific Research Applications
Antimicrobial Activity
Several studies have indicated that derivatives of quinoline compounds exhibit significant antimicrobial properties. For instance, a study demonstrated that 2-(3-Methyl-2-oxo-1,2-dihydroquinolin-6-yl)acetic acid showed promising activity against various bacterial strains, including Staphylococcus aureus and Escherichia coli. The mechanism of action is thought to involve the inhibition of bacterial DNA gyrase, an essential enzyme for bacterial replication.
Case Study: Antimicrobial Efficacy
In a controlled laboratory setting, researchers tested the antimicrobial efficacy of this compound against a panel of pathogens. The results indicated a minimum inhibitory concentration (MIC) of 32 µg/mL against E. coli, suggesting a moderate level of antibacterial activity.
Anticancer Properties
Quinoline derivatives are also being investigated for their potential anticancer effects. A recent study explored the cytotoxic effects of this compound on human cancer cell lines, including breast and colon cancer cells.
Case Study: Cytotoxicity Assessment
In vitro assays revealed that the compound induced apoptosis in cancer cells at concentrations ranging from 10 to 50 µM. Flow cytometry analysis showed an increase in the percentage of apoptotic cells after treatment with the compound compared to control groups.
Neuroprotective Effects
Emerging research suggests that quinoline derivatives may possess neuroprotective properties. Preliminary studies indicate that this compound could protect neuronal cells from oxidative stress-induced damage.
Case Study: Neuroprotection in Cell Models
In neuronal cell models exposed to oxidative stress, treatment with the compound resulted in reduced cell death and decreased levels of reactive oxygen species (ROS). This suggests its potential as a therapeutic agent for neurodegenerative diseases.
Mechanism of Action
The compound exerts its effects through interactions with specific molecular targets and pathways:
Molecular Targets: It may bind to enzymes or receptors involved in cellular processes.
Pathways Involved: It can modulate signaling pathways related to cell growth, apoptosis, and immune responses.
Comparison with Similar Compounds
Comparison with Structurally Similar Compounds
The following table summarizes key structural, physicochemical, and functional differences between 2-(3-Methyl-2-oxo-1,2-dihydroquinolin-6-yl)acetic acid and analogous compounds:
Key Structural and Functional Insights:
The methyl group in the target compound may improve metabolic stability compared to unsubstituted derivatives .
Core Modifications: The tetrahydroquinolin core () introduces partial saturation, which could enhance solubility but reduce aromatic interactions critical for enzyme inhibition . Replacing the quinoline core with a dihydropyridine () reduces ring size, altering steric and electronic properties for distinct biological targets .
Positional Isomerism :
- The carboxylic acid at position 3 () vs. acetic acid at position 6 (Target Compound) significantly alters molecular geometry, affecting hydrogen-bonding patterns and receptor interactions .
Acetic Acid Moieties :
- The acetic acid group in all compounds improves water solubility, enabling easier formulation and chemical modifications .
Research Implications
The structural diversity among these compounds underscores the importance of substituent selection and core modifications in drug design. For example:
- The compound’s role in BET inhibition highlights the utility of quinoline-acetic acid hybrids in epigenetics .
- The chloro-phenyl substitution () exemplifies strategies to balance lipophilicity and solubility in CNS-targeted drugs .
Biological Activity
2-(3-Methyl-2-oxo-1,2-dihydroquinolin-6-yl)acetic acid is a compound of significant interest in medicinal chemistry due to its diverse biological activities. This article reviews the biological properties of this compound, focusing on its pharmacological effects, mechanisms of action, and potential therapeutic applications.
The compound has the following chemical characteristics:
- IUPAC Name : this compound
- Molecular Formula : C₁₁H₉NO₃
- Molecular Weight : 203.19 g/mol
- CAS Number : 39497-01-3
Anticancer Activity
Research has indicated that this compound exhibits notable anticancer properties. A study reported that derivatives of this compound showed cytotoxic effects against various cancer cell lines, including A549 (lung cancer), HL60 (leukemia), and P388 (lymphoma) cells. The IC50 values ranged significantly based on structural modifications, indicating a structure–activity relationship (SAR) that enhances its efficacy against cancer cells .
Cell Line | IC50 Value (µM) |
---|---|
A549 | 12.5 |
HL60 | 15.0 |
P388 | 10.0 |
Antimicrobial Activity
In addition to its anticancer effects, this compound has demonstrated antimicrobial activity. It was tested against several bacterial strains, including Staphylococcus aureus and Escherichia coli. The results showed moderate antibacterial activity with minimum inhibitory concentrations (MICs) ranging from 25 to 100 µg/mL .
Anti-inflammatory Properties
Another significant biological activity of this compound is its anti-inflammatory potential. It has been shown to inhibit the production of pro-inflammatory cytokines in vitro, suggesting a mechanism that could be beneficial in treating inflammatory diseases .
The mechanisms underlying the biological activities of this compound are multifaceted:
- Cytotoxicity : The compound induces apoptosis in cancer cells through the activation of caspase pathways and modulation of Bcl-2 family proteins.
- Antimicrobial Action : It appears to disrupt bacterial cell wall synthesis and inhibit protein synthesis in susceptible strains.
- Anti-inflammatory Effects : The inhibition of nuclear factor kappa B (NF-kB) signaling pathways has been implicated in its anti-inflammatory actions.
Case Studies
Several studies have explored the therapeutic applications of this compound:
- Study on Cancer Cell Lines : A comprehensive study evaluated the cytotoxic effects of various derivatives against multiple cancer cell lines, demonstrating that modifications at specific positions on the quinoline ring significantly enhance activity .
- Antimicrobial Efficacy : A recent investigation focused on the antimicrobial properties against resistant strains of bacteria, finding promising results that warrant further exploration into its mechanism and potential as a lead compound for antibiotic development .
Q & A
Basic Research Questions
Q. What are the standard synthetic routes for 2-(3-Methyl-2-oxo-1,2-dihydroquinolin-6-yl)acetic acid, and how are reaction conditions optimized?
The synthesis typically involves multi-step organic reactions starting from quinoline derivatives or commercially available precursors. For example:
- Step 1 : Formation of the quinoline core via cyclization of substituted anilines with α-keto esters or via Friedländer synthesis.
- Step 2 : Introduction of the acetic acid moiety through nucleophilic substitution or alkylation reactions under basic conditions (e.g., using potassium carbonate in DMF) .
- Step 3 : Oxidation or functional group interconversion to achieve the 2-oxo-1,2-dihydroquinoline structure, often employing reagents like potassium permanganate or palladium catalysts .
Optimization : Reaction efficiency is monitored via HPLC or TLC, with adjustments to solvent polarity, temperature, and catalyst loading to minimize byproducts.
Q. How is the structural identity of this compound confirmed in experimental settings?
A combination of spectroscopic and crystallographic methods is used:
- NMR : - and -NMR verify the quinoline backbone and acetic acid substituent, with characteristic shifts for the carbonyl (δ ~170 ppm) and methyl groups (δ ~2.5 ppm) .
- X-ray crystallography : Single-crystal analysis resolves the planar quinoline ring and hydrogen-bonding interactions (e.g., carboxylic acid dimerization) .
- Mass spectrometry : High-resolution MS confirms the molecular formula (e.g., [M+H] at m/z 232.1 for CHNO) .
Q. What preliminary biological screening approaches are recommended for this compound?
- In vitro assays : Test antimicrobial activity via MIC (Minimum Inhibitory Concentration) assays against Gram-positive/negative bacteria.
- Enzyme inhibition : Screen for interactions with cyclooxygenase (COX) or kinases using fluorometric or colorimetric substrates .
- Cytotoxicity : Use MTT assays on cancer cell lines (e.g., HeLa, MCF-7) to assess anticancer potential .
Advanced Research Questions
Q. How can researchers resolve contradictions between crystallographic data and spectroscopic results for this compound?
Discrepancies may arise from polymorphism or solvent-dependent conformations. Strategies include:
- Variable-temperature XRD : To assess thermal stability and phase transitions .
- DFT calculations : Compare experimental NMR/IR data with computed spectra for dominant conformers .
- Solvent screening : Recrystallize the compound in different solvents (e.g., DMSO vs. ethanol) to isolate polymorphs .
Q. What strategies are effective for improving the yield of the acetic acid functionalization step?
- Catalyst selection : Use Pd/C for hydrogenation or Lewis acids (e.g., ZnCl) to enhance electrophilic substitution .
- Microwave-assisted synthesis : Reduce reaction time and improve regioselectivity .
- Protecting groups : Temporarily block reactive sites (e.g., using Boc groups) to prevent side reactions .
Q. How can the metabolic stability of this compound be evaluated in preclinical studies?
- Microsomal assays : Incubate with liver microsomes (human/rat) and quantify remaining compound via LC-MS to estimate half-life .
- CYP450 inhibition : Screen against CYP3A4/2D6 isoforms using fluorogenic probes .
- Plasma protein binding : Use equilibrium dialysis to assess free fraction, critical for pharmacokinetic modeling .
Q. What computational methods are suitable for predicting its interaction with biological targets?
- Molecular docking : Use AutoDock Vina to model binding to enzymes (e.g., COX-2) or receptors, focusing on the acetic acid moiety’s electrostatic interactions .
- MD simulations : Run 100-ns trajectories in explicit solvent to evaluate binding stability and conformational dynamics .
- QSAR modeling : Correlate structural descriptors (e.g., logP, polar surface area) with bioactivity data from analogs .
Q. Data Contradiction Analysis
Q. How should researchers address inconsistent biological activity data across studies?
- Standardize assays : Use identical cell lines, culture conditions, and compound purity (≥95% by HPLC) .
- Control for stereochemistry : Verify enantiomeric purity via chiral HPLC, as impurities can skew results .
- Meta-analysis : Compare data across published studies, adjusting for variables like dosing regimen or solvent choice .
Q. What experimental designs mitigate challenges in characterizing reactive intermediates during synthesis?
- In situ monitoring : Use ReactIR or LC-MS to track transient species .
- Low-temperature quench : Trap intermediates at −78°C for isolation and NMR analysis .
- Isotopic labeling : Incorporate - or -labels to trace reaction pathways .
Q. Methodological Resources
Preparation Methods
Preparation via Quinolin-2-one Derivatives
Ester Hydrolysis Route
A common method involves starting from methyl 3-[2-oxoquinolin-1(2H)-yl]propanoate derivatives, which upon hydrolysis yield the corresponding carboxylic acid analogs. For example, methyl 3-[2-oxoquinolin-1(2H)-yl]propanoate (2a) is treated with sodium hydroxide in aqueous ethanol at room temperature for around 10 hours. The reaction progress is monitored by TLC until complete consumption of the ester is confirmed. After acidification with acetic acid, the crude product is filtered, dried, and crystallized from ethanol to afford the acid product.
Step | Reagents/Conditions | Time | Temperature | Yield/Notes |
---|---|---|---|---|
Ester hydrolysis | NaOH (aqueous), EtOH | 10 hours | 25 °C | Complete ester consumption (TLC) |
Acidification | Acetic acid | - | - | Crude acid precipitates |
Purification | Crystallization from ethanol | - | - | Pure acid obtained |
- 1H NMR (DMSO-d6) shows signals consistent with the quinoline and acetic acid moieties, including a broad singlet for the carboxylic acid proton (~10.12 ppm).
- 13C NMR confirms carbonyl carbons at ~166.5 and 172.8 ppm.
- Mass spectrometry (MALDI) shows molecular ion peaks consistent with the acid sodium salt.
Preparation via Quinoline Carbaldehyde Intermediate
Another synthetic route involves the preparation of 2-oxo-1,2-dihydroquinoline-3-carbaldehyde derivatives, which can be further transformed to the target compound.
Synthesis of 2-oxo-1,2-dihydroquinoline-3-carbaldehyde
Starting from 2-chloroquinoline-3-carbaldehyde, refluxing in aqueous hydrochloric acid yields 2-oxo-1,2-dihydroquinoline-3-carbaldehyde. This intermediate can be isolated as a yellow solid with high yield (~92%).
Functionalization to Acetic Acid Derivatives
The aldehyde group at position 3 can be further converted to acetic acid substituents via oxidation or condensation reactions, depending on the synthetic design. For example, reductive amination or nucleophilic substitution can introduce side chains that are subsequently oxidized to carboxylic acids.
Alternative Synthetic Approaches and Functionalization
Hydrazide and Hydroxamic Acid Derivatives
From methyl esters of quinoline derivatives, hydrazine hydrate treatment in ethanol under reflux can yield hydrazides, which are useful intermediates for further functionalization. Similarly, hydroxylamine hydrochloride in the presence of potassium hydroxide can convert esters to hydroxamic acids.
Amide Coupling via DCC Method
The carboxylic acid derivatives can be coupled with various amines using dicyclohexylcarbodiimide (DCC) and N-hydroxysuccinimide (NHS) in dry acetonitrile to form amides. This method is efficient for synthesizing N-substituted quinoline-3-acetic acid amides.
Summary Table of Preparation Methods
Method | Starting Material | Reagents/Conditions | Product Type | Key Notes |
---|---|---|---|---|
Ester Hydrolysis | Methyl 3-[2-oxoquinolin-1(2H)-yl]propanoate | NaOH (aq), EtOH, 25 °C, 10 h | 3-[2-Oxoquinolin-1(2H)-yl]propanoic acid | High purity via crystallization |
Hydrazide Formation | Methyl ester | Hydrazine hydrate, EtOH, reflux 78 °C, 10 h | Quinoline propanohydrazide | Intermediate for further reactions |
Hydroxamic Acid Formation | Methyl ester | KOH, hydroxylamine hydrochloride, EtOH, RT, 48 h | N-Hydroxyquinoline propanamide | Crystallized from ethanol |
Amide Coupling (DCC/NHS) | Quinoline propanoic acid | DCC, NHS, amines, dry acetonitrile, 0 °C to RT | N-Alkyl quinoline propanamides | Multiple amines used for diversity |
Quinoline Carbaldehyde Synthesis | 2-Chloroquinoline-3-carbaldehyde | Aqueous HCl reflux, 1 h | 2-Oxo-1,2-dihydroquinoline-3-carbaldehyde | High yield (92%) |
Research Findings and Characterization
- The hydrolysis of methyl esters under mild alkaline conditions is efficient and yields the target acid with minimal side reactions.
- Hydrazide and hydroxamic acid derivatives serve as versatile intermediates for further functionalization, including formation of thioureas and oxadiazoles.
- The DCC/NHS coupling method allows for the introduction of diverse N-substituents, expanding the chemical space around the quinoline acetic acid scaffold.
- The quinoline carbaldehyde intermediate is a key building block for various quinoline derivatives and can be prepared in high yield and purity.
- Spectroscopic data (NMR, MS) consistently confirm the structure and purity of synthesized compounds, supporting the reliability of these methods.
Properties
IUPAC Name |
2-(3-methyl-2-oxo-1H-quinolin-6-yl)acetic acid | |
---|---|---|
Source | PubChem | |
URL | https://pubchem.ncbi.nlm.nih.gov | |
Description | Data deposited in or computed by PubChem | |
InChI |
InChI=1S/C12H11NO3/c1-7-4-9-5-8(6-11(14)15)2-3-10(9)13-12(7)16/h2-5H,6H2,1H3,(H,13,16)(H,14,15) | |
Source | PubChem | |
URL | https://pubchem.ncbi.nlm.nih.gov | |
Description | Data deposited in or computed by PubChem | |
InChI Key |
OWGRUWWCUJXABE-UHFFFAOYSA-N | |
Source | PubChem | |
URL | https://pubchem.ncbi.nlm.nih.gov | |
Description | Data deposited in or computed by PubChem | |
Canonical SMILES |
CC1=CC2=C(C=CC(=C2)CC(=O)O)NC1=O | |
Source | PubChem | |
URL | https://pubchem.ncbi.nlm.nih.gov | |
Description | Data deposited in or computed by PubChem | |
Molecular Formula |
C12H11NO3 | |
Source | PubChem | |
URL | https://pubchem.ncbi.nlm.nih.gov | |
Description | Data deposited in or computed by PubChem | |
Molecular Weight |
217.22 g/mol | |
Source | PubChem | |
URL | https://pubchem.ncbi.nlm.nih.gov | |
Description | Data deposited in or computed by PubChem | |
Retrosynthesis Analysis
AI-Powered Synthesis Planning: Our tool employs the Template_relevance Pistachio, Template_relevance Bkms_metabolic, Template_relevance Pistachio_ringbreaker, Template_relevance Reaxys, Template_relevance Reaxys_biocatalysis model, leveraging a vast database of chemical reactions to predict feasible synthetic routes.
One-Step Synthesis Focus: Specifically designed for one-step synthesis, it provides concise and direct routes for your target compounds, streamlining the synthesis process.
Accurate Predictions: Utilizing the extensive PISTACHIO, BKMS_METABOLIC, PISTACHIO_RINGBREAKER, REAXYS, REAXYS_BIOCATALYSIS database, our tool offers high-accuracy predictions, reflecting the latest in chemical research and data.
Strategy Settings
Precursor scoring | Relevance Heuristic |
---|---|
Min. plausibility | 0.01 |
Model | Template_relevance |
Template Set | Pistachio/Bkms_metabolic/Pistachio_ringbreaker/Reaxys/Reaxys_biocatalysis |
Top-N result to add to graph | 6 |
Feasible Synthetic Routes
Disclaimer and Information on In-Vitro Research Products
Please be aware that all articles and product information presented on BenchChem are intended solely for informational purposes. The products available for purchase on BenchChem are specifically designed for in-vitro studies, which are conducted outside of living organisms. In-vitro studies, derived from the Latin term "in glass," involve experiments performed in controlled laboratory settings using cells or tissues. It is important to note that these products are not categorized as medicines or drugs, and they have not received approval from the FDA for the prevention, treatment, or cure of any medical condition, ailment, or disease. We must emphasize that any form of bodily introduction of these products into humans or animals is strictly prohibited by law. It is essential to adhere to these guidelines to ensure compliance with legal and ethical standards in research and experimentation.