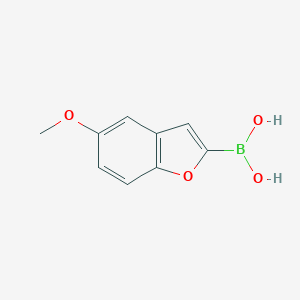
(5-Methoxybenzofuran-2-yl)boronic acid
Overview
Description
(5-Methoxybenzofuran-2-yl)boronic acid is an organic compound with the molecular formula C9H9BO4 It is a boronic acid derivative of benzofuran, characterized by the presence of a methoxy group at the 5-position of the benzofuran ring
Mechanism of Action
Target of Action
The primary target of (5-Methoxybenzofuran-2-yl)boronic acid is the palladium catalyst in the Suzuki–Miyaura (SM) cross-coupling reaction . This reaction is a widely-applied transition metal catalysed carbon–carbon bond forming reaction .
Mode of Action
The compound interacts with its target through a process known as transmetalation . In this process, the boronic acid group of the compound is transferred from boron to palladium . This is a key step in the SM cross-coupling reaction .
Biochemical Pathways
The SM cross-coupling reaction is the primary biochemical pathway affected by this compound . The reaction conjoins chemically differentiated fragments that participate in electronically divergent processes with the metal catalyst . The downstream effects of this pathway include the formation of new carbon-carbon bonds .
Pharmacokinetics
It is known that the compound has high gi absorption . The compound’s ADME properties and their impact on bioavailability would need further investigation.
Result of Action
The result of the compound’s action is the formation of new carbon-carbon bonds through the SM cross-coupling reaction . This can lead to the synthesis of a wide range of organic compounds .
Action Environment
The action of this compound is influenced by several environmental factors. For instance, the reaction conditions for the SM cross-coupling reaction are exceptionally mild and functional group tolerant . Additionally, the compound is relatively stable, readily prepared, and generally environmentally benign . The slow release rate of the active boronic acid allows it to stay in low concentration, which leads to a favorable partitioning between cross-coupling and oxidative homo-coupling .
Preparation Methods
Synthetic Routes and Reaction Conditions
The synthesis of (5-Methoxybenzofuran-2-yl)boronic acid typically involves the lithiation of 5-methoxybenzofuran followed by borylation. One common method includes the following steps:
Lithiation: 5-Methoxybenzofuran is treated with n-butyllithium in tetrahydrofuran (THF) at -78°C to form the lithiated intermediate.
Borylation: The lithiated intermediate is then reacted with trimethyl borate at -30°C to 10°C.
Hydrolysis: The resulting boronate ester is hydrolyzed with hydrochloric acid to yield this compound.
Industrial Production Methods
While specific industrial production methods for this compound are not widely documented, the general approach involves scaling up the laboratory synthesis procedures. This includes optimizing reaction conditions, using larger reactors, and ensuring proper handling and storage conditions to maintain the purity and stability of the compound.
Chemical Reactions Analysis
Types of Reactions
(5-Methoxybenzofuran-2-yl)boronic acid undergoes various chemical reactions, including:
Suzuki-Miyaura Coupling: This reaction involves the coupling of this compound with aryl or vinyl halides in the presence of a palladium catalyst and a base, forming carbon-carbon bonds.
Oxidation: The compound can be oxidized to form corresponding phenols or quinones.
Substitution: Electrophilic substitution reactions can occur at the benzofuran ring, particularly at the 2-position.
Common Reagents and Conditions
Suzuki-Miyaura Coupling: Palladium catalysts (e.g., Pd(PPh3)4), bases (e.g., K2CO3), and solvents (e.g., toluene, ethanol).
Oxidation: Oxidizing agents such as hydrogen peroxide or potassium permanganate.
Substitution: Electrophiles such as halogens or nitro groups under acidic or basic conditions.
Major Products
Suzuki-Miyaura Coupling: Biaryl compounds.
Oxidation: Phenols or quinones.
Substitution: Halogenated or nitro-substituted benzofuran derivatives.
Scientific Research Applications
(5-Methoxybenzofuran-2-yl)boronic acid has diverse applications in scientific research:
Chemistry: Used as a building block in organic synthesis, particularly in the formation of biaryl compounds through Suzuki-Miyaura coupling.
Biology: Investigated for its potential as a pharmacophore in drug design and development.
Medicine: Explored for its potential therapeutic properties, including anti-inflammatory and anticancer activities.
Industry: Utilized in the synthesis of advanced materials and polymers.
Comparison with Similar Compounds
Similar Compounds
- (5-Methylbenzofuran-2-yl)boronic acid
- (5-Bromobenzofuran-2-yl)boronic acid
- (5-Fluorobenzofuran-2-yl)boronic acid
- (5-Chlorobenzofuran-2-yl)boronic acid
Uniqueness
(5-Methoxybenzofuran-2-yl)boronic acid is unique due to the presence of the methoxy group, which can enhance its solubility and influence its electronic properties. This makes it particularly useful in certain synthetic applications where electronic effects play a crucial role in the reactivity and selectivity of the compound .
Properties
IUPAC Name |
(5-methoxy-1-benzofuran-2-yl)boronic acid | |
---|---|---|
Source | PubChem | |
URL | https://pubchem.ncbi.nlm.nih.gov | |
Description | Data deposited in or computed by PubChem | |
InChI |
InChI=1S/C9H9BO4/c1-13-7-2-3-8-6(4-7)5-9(14-8)10(11)12/h2-5,11-12H,1H3 | |
Source | PubChem | |
URL | https://pubchem.ncbi.nlm.nih.gov | |
Description | Data deposited in or computed by PubChem | |
InChI Key |
XLIQZZGLIJLKTF-UHFFFAOYSA-N | |
Source | PubChem | |
URL | https://pubchem.ncbi.nlm.nih.gov | |
Description | Data deposited in or computed by PubChem | |
Canonical SMILES |
B(C1=CC2=C(O1)C=CC(=C2)OC)(O)O | |
Source | PubChem | |
URL | https://pubchem.ncbi.nlm.nih.gov | |
Description | Data deposited in or computed by PubChem | |
Molecular Formula |
C9H9BO4 | |
Source | PubChem | |
URL | https://pubchem.ncbi.nlm.nih.gov | |
Description | Data deposited in or computed by PubChem | |
DSSTOX Substance ID |
DTXSID10623527 | |
Record name | (5-Methoxy-1-benzofuran-2-yl)boronic acid | |
Source | EPA DSSTox | |
URL | https://comptox.epa.gov/dashboard/DTXSID10623527 | |
Description | DSSTox provides a high quality public chemistry resource for supporting improved predictive toxicology. | |
Molecular Weight |
191.98 g/mol | |
Source | PubChem | |
URL | https://pubchem.ncbi.nlm.nih.gov | |
Description | Data deposited in or computed by PubChem | |
CAS No. |
551001-79-7 | |
Record name | (5-Methoxy-1-benzofuran-2-yl)boronic acid | |
Source | EPA DSSTox | |
URL | https://comptox.epa.gov/dashboard/DTXSID10623527 | |
Description | DSSTox provides a high quality public chemistry resource for supporting improved predictive toxicology. | |
Synthesis routes and methods I
Procedure details
Synthesis routes and methods II
Procedure details
Synthesis routes and methods III
Procedure details
Retrosynthesis Analysis
AI-Powered Synthesis Planning: Our tool employs the Template_relevance Pistachio, Template_relevance Bkms_metabolic, Template_relevance Pistachio_ringbreaker, Template_relevance Reaxys, Template_relevance Reaxys_biocatalysis model, leveraging a vast database of chemical reactions to predict feasible synthetic routes.
One-Step Synthesis Focus: Specifically designed for one-step synthesis, it provides concise and direct routes for your target compounds, streamlining the synthesis process.
Accurate Predictions: Utilizing the extensive PISTACHIO, BKMS_METABOLIC, PISTACHIO_RINGBREAKER, REAXYS, REAXYS_BIOCATALYSIS database, our tool offers high-accuracy predictions, reflecting the latest in chemical research and data.
Strategy Settings
Precursor scoring | Relevance Heuristic |
---|---|
Min. plausibility | 0.01 |
Model | Template_relevance |
Template Set | Pistachio/Bkms_metabolic/Pistachio_ringbreaker/Reaxys/Reaxys_biocatalysis |
Top-N result to add to graph | 6 |
Feasible Synthetic Routes
Disclaimer and Information on In-Vitro Research Products
Please be aware that all articles and product information presented on BenchChem are intended solely for informational purposes. The products available for purchase on BenchChem are specifically designed for in-vitro studies, which are conducted outside of living organisms. In-vitro studies, derived from the Latin term "in glass," involve experiments performed in controlled laboratory settings using cells or tissues. It is important to note that these products are not categorized as medicines or drugs, and they have not received approval from the FDA for the prevention, treatment, or cure of any medical condition, ailment, or disease. We must emphasize that any form of bodily introduction of these products into humans or animals is strictly prohibited by law. It is essential to adhere to these guidelines to ensure compliance with legal and ethical standards in research and experimentation.