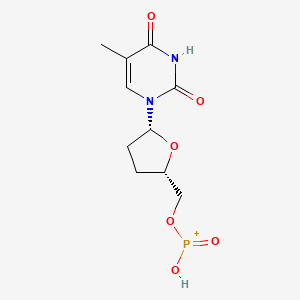
ddT-HP
- Click on QUICK INQUIRY to receive a quote from our team of experts.
- With the quality product at a COMPETITIVE price, you can focus more on your research.
Overview
Description
The compound “ddT-HP” is a synthetic chemical known for its insecticidal properties. It belongs to the class of organochlorine compounds and has been widely used in agriculture and public health for controlling pests and vector-borne diseases. The compound is colorless, tasteless, and almost odorless, making it suitable for various applications.
Preparation Methods
Synthetic Routes and Reaction Conditions
The synthesis of “ddT-HP” involves the condensation of chloral hydrate with chlorobenzene in the presence of a catalyst. The reaction typically occurs under reflux conditions, and the product is purified through recrystallization. The reaction can be represented as follows:
[ \text{CCl}_3\text{CHO} + 2 \text{C}_6\text{H}_5\text{Cl} \rightarrow \text{C}_6\text{H}_4\text{Cl}_2\text{CCl}_3 + \text{H}_2\text{O} ]
Industrial Production Methods
In industrial settings, the production of “this compound” is carried out in large reactors with precise control over temperature and pressure. The process involves continuous monitoring and optimization to ensure high yield and purity. The final product is subjected to rigorous quality control tests before being packaged for distribution.
Chemical Reactions Analysis
Types of Reactions
“ddT-HP” undergoes various chemical reactions, including:
Oxidation: The compound can be oxidized to form dichlorodiphenyldichloroethylene (DDE) and dichlorodiphenyldichloroethane (DDD).
Reduction: Reduction reactions can convert “this compound” to less chlorinated derivatives.
Substitution: Halogen substitution reactions can occur, leading to the formation of different chlorinated compounds.
Common Reagents and Conditions
Oxidation: Common oxidizing agents include potassium permanganate and hydrogen peroxide.
Reduction: Reducing agents such as sodium borohydride and lithium aluminum hydride are used.
Substitution: Halogen exchange reactions can be facilitated by reagents like sodium iodide in acetone.
Major Products Formed
Oxidation: Dichlorodiphenyldichloroethylene (DDE) and dichlorodiphenyldichloroethane (DDD).
Reduction: Less chlorinated derivatives of “this compound”.
Substitution: Various chlorinated compounds depending on the halogen used.
Scientific Research Applications
“ddT-HP” has been extensively studied for its applications in various fields:
Chemistry: Used as a model compound for studying organochlorine chemistry and reaction mechanisms.
Biology: Investigated for its effects on insect physiology and resistance mechanisms.
Medicine: Explored for potential therapeutic applications in treating vector-borne diseases.
Industry: Utilized in the production of pesticides and insect repellents.
Mechanism of Action
The mechanism of action of “ddT-HP” involves its interaction with the nervous system of insects. The compound disrupts the normal functioning of sodium channels in nerve cells, leading to uncontrolled nerve impulses and eventual paralysis of the insect. This mode of action makes “this compound” highly effective as an insecticide.
Comparison with Similar Compounds
Similar Compounds
Dichlorodiphenyltrichloroethane (DDT): A well-known insecticide with similar chemical structure and properties.
Dichlorodiphenyldichloroethylene (DDE): A degradation product of “ddT-HP” with similar insecticidal properties.
Dichlorodiphenyldichloroethane (DDD): Another degradation product with comparable effects.
Uniqueness
“this compound” is unique due to its high stability and persistence in the environment. Unlike some of its degradation products, “this compound” retains its insecticidal properties for extended periods, making it highly effective in long-term pest control.
Properties
CAS No. |
140132-19-0 |
---|---|
Molecular Formula |
C10H14N2O6P+ |
Molecular Weight |
289.20 g/mol |
IUPAC Name |
hydroxy-[[(2S,5R)-5-(5-methyl-2,4-dioxopyrimidin-1-yl)oxolan-2-yl]methoxy]-oxophosphanium |
InChI |
InChI=1S/C10H13N2O6P/c1-6-4-12(10(14)11-9(6)13)8-3-2-7(18-8)5-17-19(15)16/h4,7-8H,2-3,5H2,1H3,(H-,11,13,14,15,16)/p+1/t7-,8+/m0/s1 |
InChI Key |
NDGHBKNMUFNTFA-JGVFFNPUSA-O |
Isomeric SMILES |
CC1=CN(C(=O)NC1=O)[C@H]2CC[C@H](O2)CO[P+](=O)O |
Canonical SMILES |
CC1=CN(C(=O)NC1=O)C2CCC(O2)CO[P+](=O)O |
Origin of Product |
United States |
Disclaimer and Information on In-Vitro Research Products
Please be aware that all articles and product information presented on BenchChem are intended solely for informational purposes. The products available for purchase on BenchChem are specifically designed for in-vitro studies, which are conducted outside of living organisms. In-vitro studies, derived from the Latin term "in glass," involve experiments performed in controlled laboratory settings using cells or tissues. It is important to note that these products are not categorized as medicines or drugs, and they have not received approval from the FDA for the prevention, treatment, or cure of any medical condition, ailment, or disease. We must emphasize that any form of bodily introduction of these products into humans or animals is strictly prohibited by law. It is essential to adhere to these guidelines to ensure compliance with legal and ethical standards in research and experimentation.