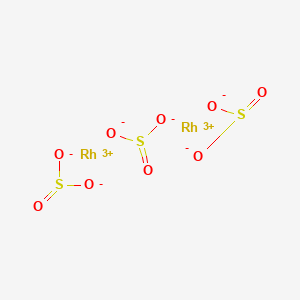
Dirhodium trisulphite
- Click on QUICK INQUIRY to receive a quote from our team of experts.
- With the quality product at a COMPETITIVE price, you can focus more on your research.
Overview
Description
Dirhodium complexes represent a critical class of bimetallic compounds with diverse catalytic and biomedical applications. These complexes exhibit unique structural and reactive properties due to their Rh–Rh bonding and ligand coordination modes. For example, dirhodium tetraacetate ([Rh₂(OAc)₄]) demonstrates axial coordination to biological macromolecules like DNA , while dirhodium tetraphosphine complexes (Rh₂(nbd)₂(rac-et,ph-P4)₂) are notable for their role in hydroformylation catalysis, albeit with solvent-dependent fragmentation .
Preparation Methods
The synthesis of dirhodium trisulphite typically involves the reaction of rhodium salts with sulfur-containing reagents under controlled conditions. One common method involves the use of rhodium(III) chloride and sodium sulfide in an aqueous solution, followed by precipitation and purification steps . The reaction conditions, such as temperature, pH, and concentration, play a crucial role in determining the yield and purity of the final product . Industrial production methods may involve similar synthetic routes but on a larger scale, with additional steps for purification and quality control to ensure consistency and reproducibility .
Chemical Reactions Analysis
Related Rhodium-Sulfur Compounds
-
Dirhodium Trisulphate (Rh₂(SO₄)₃)
-
Dirhodium Trisulphide (Rh₂S₃)
Rhodium-Catalyzed Reactions Involving Sulfur Compounds
While no data exists for dirhodium trisulphite, rhodium complexes are known to catalyze reactions with organosulfur compounds:
Disulfide Exchange Reactions
-
Rhodium complexes (e.g., RhH(PPh₃)₄) catalyze S-S bond cleavage and organothio group transfer:
Thiols and Disulfide Interconversion
-
Rhodium catalysts enable reversible oxidation/reduction of thiols and disulfides:
Structural and Mechanistic Insights
Scientific Research Applications
Dirhodium trisulphite has a wide range of scientific research applications, particularly in the fields of chemistry, biology, medicine, and industry. In chemistry, it is used as a catalyst for various organic transformations, including cycloisomerization, hetero-Diels–Alder reactions, ene reactions, arylation, radical oxidation reactions, and C–H activation . In biology and medicine, dirhodium compounds have shown potential as anticancer agents due to their ability to inhibit the ubiquitin-proteasome system, which is crucial for protein degradation and cell cycle regulation . Additionally, this compound is explored for its potential use in industrial processes, such as the selective reduction of CO₂ to HCOOH and proton reduction to generate hydrogen fuel .
Mechanism of Action
The mechanism of action of dirhodium trisulphite involves its interaction with molecular targets and pathways within cells. In the context of its anticancer properties, this compound inhibits the ubiquitin-proteasome system, leading to the accumulation of ubiquitinated proteins and disruption of cellular homeostasis . This inhibition is achieved through the binding of this compound to the active sites of proteasomal enzymes, thereby preventing the degradation of target proteins . Additionally, this compound can catalyze the formation of reactive intermediates, such as carbenes and nitrenes, which can further interact with cellular components and induce cytotoxic effects .
Comparison with Similar Compounds
Dirhodium Tetraacetate vs. Cisplatin (DNA Binding)
Property | Dirhodium Tetraacetate | Cisplatin |
---|---|---|
Binding Site | Axial coordination to adenine (Ade5) | Equatorial binding to guanine (Gua4, Gua10, Gua16) |
Coordination Mode | Prefers N-atom axial interactions | Crosslinks DNA via Pt–N7 guanine bonds |
Structural Impact | Minimal distortion of DNA helix | Significant helix distortion |
Biological Target | Adenine-rich sequences | Guanine-rich regions |
X-ray crystallography and mass spectrometry reveal that dirhodium tetraacetate binds selectively to adenine residues in a B-DNA dodecamer, unlike cisplatin, which primarily targets guanine . This distinction suggests dirhodium complexes may avoid common cisplatin resistance mechanisms tied to guanine adduct repair.
Dirhodium vs. Diruthenium Complexes (Protein Interactions)
Dirhodium and diruthenium compounds exhibit contrasting preferences in protein coordination:
- Dirhodium Tetraacetate : Favors axial coordination to histidine or cysteine residues in proteins (e.g., cytochrome c, lysozyme) .
- Diruthenium Complexes : Prefer equatorial binding, often forming stable adducts with carboxylate or amine groups .
This axial vs. equatorial dichotomy influences their pharmacokinetics and toxicity profiles, making dirhodium compounds more suitable for targeting specific nucleic acid motifs.
Catalytic Dirhodium Complexes: Tetraphosphine vs. Carboxylates
Dirhodium catalysts vary widely in stability and activity depending on ligand architecture:
Dirhodium carboxylates, such as Rh₂(esp)₂, are superior for asymmetric synthesis due to their robustness, whereas tetraphosphine complexes require stringent solvent conditions to prevent decomposition .
Comparison with Sodium Trisulphite (Anionic Ligand Systems)
While sodium trisulphite (Na₂S₃O₆) is an inorganic sulfite salt, dirhodium complexes with sulfite ligands remain underexplored. Hypothetically, a "dirhodium trisulphite" complex might exhibit:
- Redox Activity : Sulfite ligands could enable redox-switchable catalysis.
- Toxicity Profile : Likely lower acute toxicity compared to cisplatin or dirhodium acetate, based on sulfite’s moderate hazard classification (see sodium trisulphite safety data ).
Data Tables for Key Comparisons
Table 1: DNA-Binding Properties of Metallodrugs
Compound | Binding Site | Coordination Mode | Structural Impact |
---|---|---|---|
Dirhodium Tetraacetate | Ade5 | Axial N-coordination | Minimal distortion |
Cisplatin | Gua4, Gua10, Gua16 | Equatorial Pt–N7 | Helix kinking |
Table 2: Catalytic Performance of Dirhodium Complexes
Catalyst | Application | Turnover Frequency (TOF) | Solvent Stability |
---|---|---|---|
Dirhodium Carboxylate | C–H Insertion | >1,000 h⁻¹ | High |
Dirhodium Tetraphosphine | Hydroformylation | ~200 h⁻¹ | Low (fragments in acetone) |
Biological Activity
Dirhodium trisulphite, a compound featuring rhodium in its coordination with sulfur, has gained attention for its potential biological activity, particularly in catalyzing reactions involving organosulfur compounds. This article explores the biological activity of this compound, focusing on its mechanisms, applications, and relevant case studies.
Overview of this compound
This compound (Rh2(SO3)3) is a transition metal complex that has been studied for its catalytic properties in organic synthesis, particularly in reactions involving sulfur-containing compounds. Rhodium complexes are known for their ability to facilitate various chemical transformations, including oxidation and reduction processes.
Rhodium catalysts, including this compound, operate through several mechanisms that enhance the reactivity of sulfur compounds. The primary mechanisms include:
- Catalytic Activation : Rhodium can activate disulfides and thiols, facilitating their transformation into more reactive species. This is crucial in biological systems where sulfur-containing amino acids play a significant role.
- Redox Reactions : this compound can mediate redox reactions involving sulfur species, which are essential in biochemical pathways such as detoxification and metabolism.
- Selective Functionalization : The ability to selectively functionalize sulfur compounds allows for the modification of biomolecules, potentially enhancing their biological activity or altering their interactions with other cellular components.
Biological Applications
This compound's biological activity has been explored in various contexts:
- Modification of Peptides and Proteins : Rhodium-catalyzed reactions enable the modification of cysteine residues in peptides and proteins without the need for protecting groups. This is significant for studying protein function and interactions in biological systems .
- Synthesis of Organosulfur Compounds : The compound has been utilized in synthesizing biologically active organosulfur compounds, which have implications in medicinal chemistry and drug development .
Case Studies
- Rhodium-Catalyzed Synthesis of Methylthiolated Glutathione :
- Disulfide Exchange Reactions :
- Thiol Reduction :
Data Table: Summary of Biological Activities
Activity | Description | Yield/Outcome |
---|---|---|
Methylthiolation of Glutathione | Modification of glutathione disulfide using RhCl₃ | 81% yield |
Disulfide Exchange | Catalysis of disulfide exchange reactions | High functional group tolerance |
Thiol Reduction | Conversion of disulfides to thiols | 93% yield (octanethiol) |
Q & A
Basic Research Questions
Q. What are the established methods for synthesizing and characterizing dirhodium trisulphite in laboratory settings?
- Methodological Answer : this compound is typically synthesized via ligand substitution reactions, starting from dirhodium tetraacetate ([Rh₂(μ-O₂CCH₃)₄]) and replacing acetate ligands with sulphite groups under controlled pH and temperature conditions. Characterization involves X-ray crystallography to confirm molecular geometry, nuclear magnetic resonance (NMR) to analyze ligand coordination modes, and electrospray ionization mass spectrometry (ESI-MS) to verify molecular weight and purity .
Q. Which analytical techniques are most reliable for studying the coordination chemistry of this compound with biological molecules?
- Methodological Answer : Key techniques include:
- X-ray crystallography to resolve atomic-level binding interactions (e.g., axial vs. equatorial coordination to purine bases) .
- Bidimensional NMR to map hydrogen bonding and ligand dynamics in solution .
- ESI-MS to detect adduct formation with DNA oligonucleotides or proteins .
Q. What are the primary biological targets of this compound, and how do these interactions compare to other metallodrugs like cisplatin?
- Methodological Answer : this compound primarily targets purine bases in DNA, with preferential axial binding to adenine (N7/N1) and guanine (N7), stabilized by hydrogen bonding with NH₂ groups. Unlike cisplatin, which forms covalent crosslinks with guanine (e.g., Gua4, Gua10 in dodecamers), this compound exhibits distinct binding patterns, favoring monodentate coordination rather than crosslinking. Comparative studies require parallel crystallographic and mass spectrometry analyses of DNA adducts .
Advanced Research Questions
Q. How can researchers design experiments to resolve contradictions in reported binding modes (axial vs. equatorial) of this compound with DNA?
- Methodological Answer : To address discrepancies:
- Combine X-ray crystallography (for static binding snapshots) with solution-phase NMR to assess dynamic equilibria between axial and equatorial coordination.
- Use competitive binding assays with synthetic oligonucleotides containing modified bases (e.g., methylated adenine) to probe steric and electronic influences.
- Validate findings with density functional theory (DFT) calculations to model energy differences between binding modes .
Q. What experimental frameworks are optimal for studying the reactivity of this compound with non-canonical DNA structures (e.g., G-quadruplexes or mismatched duplexes)?
- Methodological Answer :
- Circular dichroism (CD) and thermal denaturation assays to monitor structural changes in DNA upon dirhodium binding.
- Single-crystal X-ray diffraction of co-crystallized dirhodium-G-quadruplex complexes.
- Fluorescence-based assays with labeled oligonucleotides to quantify binding kinetics under varying ionic conditions .
Q. How can researchers reconcile conflicting literature on the preference of this compound for adenine vs. guanine in different experimental models?
- Methodological Answer :
- Conduct sequence-specific binding studies using defined oligonucleotides (e.g., C-G-C-G-A-A-T-T-C-G-C-G) to isolate base preferences.
- Compare results across techniques: crystallography (solid-state bias) vs. NMR (solution-phase dynamics).
- Analyze pH-dependent binding behavior, as protonation states of NH₂ groups in adenine/guanine may influence coordination .
Q. What strategies are effective for evaluating the impact of ligand substitution (e.g., sulphite vs. acetate) on the stability and reactivity of dirhodium complexes?
- Methodological Answer :
- Perform ligand-exchange kinetics studies using stopped-flow spectrophotometry.
- Compare redox potentials via cyclic voltammetry to assess ligand influence on dirhodium’s electronic structure.
- Use spectroscopic titrations (UV-Vis, IR) to monitor ligand displacement in real-time .
Q. How should researchers approach stability testing of this compound under physiological conditions (e.g., pH, temperature, serum proteins)?
- Methodological Answer :
- Use accelerated stability testing in buffers mimicking physiological pH (7.4) and temperature (37°C).
- Employ size-exclusion chromatography or dynamic light scattering (DLS) to detect aggregation or decomposition.
- Conduct competitive binding assays with serum albumin to evaluate protein interference .
Q. Methodological Frameworks for Research Design
- For feasibility : Apply the SMART framework (Specific, Measurable, Achievable, Researchable, Time-bound) to define scope, e.g., limiting binding studies to adenine-rich sequences .
- For novelty : Use the FINER criteria (Feasible, Interesting, Novel, Ethical, Relevant) to prioritize questions, such as comparing this compound’s mechanism to understudied metallodrugs .
Properties
CAS No. |
80048-77-7 |
---|---|
Molecular Formula |
O9Rh2S3 |
Molecular Weight |
446.0 g/mol |
IUPAC Name |
rhodium(3+);trisulfite |
InChI |
InChI=1S/3H2O3S.2Rh/c3*1-4(2)3;;/h3*(H2,1,2,3);;/q;;;2*+3/p-6 |
InChI Key |
PJRGNVSDUQPLCM-UHFFFAOYSA-H |
Canonical SMILES |
[O-]S(=O)[O-].[O-]S(=O)[O-].[O-]S(=O)[O-].[Rh+3].[Rh+3] |
Origin of Product |
United States |
Disclaimer and Information on In-Vitro Research Products
Please be aware that all articles and product information presented on BenchChem are intended solely for informational purposes. The products available for purchase on BenchChem are specifically designed for in-vitro studies, which are conducted outside of living organisms. In-vitro studies, derived from the Latin term "in glass," involve experiments performed in controlled laboratory settings using cells or tissues. It is important to note that these products are not categorized as medicines or drugs, and they have not received approval from the FDA for the prevention, treatment, or cure of any medical condition, ailment, or disease. We must emphasize that any form of bodily introduction of these products into humans or animals is strictly prohibited by law. It is essential to adhere to these guidelines to ensure compliance with legal and ethical standards in research and experimentation.