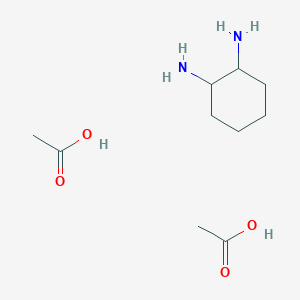
acetic acid;cyclohexane-1,2-diamine
- Click on QUICK INQUIRY to receive a quote from our team of experts.
- With the quality product at a COMPETITIVE price, you can focus more on your research.
Overview
Description
Acetic acid;cyclohexane-1,2-diamine is a compound that combines the properties of acetic acid and cyclohexane-1,2-diamine Acetic acid is a simple carboxylic acid known for its role in vinegar, while cyclohexane-1,2-diamine is a diamine with a cyclohexane backbone
Preparation Methods
Synthetic Routes and Reaction Conditions
The synthesis of acetic acid;cyclohexane-1,2-diamine can be achieved through several methods. One common approach involves the reaction of cyclohexane-1,2-diamine with acetic acid under controlled conditions. The reaction typically requires a catalyst and specific temperature and pressure settings to ensure optimal yield and purity. For example, the reaction can be carried out in the presence of a strong acid catalyst at elevated temperatures to facilitate the formation of the desired product .
Industrial Production Methods
In an industrial setting, the production of this compound may involve large-scale reactors and continuous flow systems to maximize efficiency and output. The use of advanced catalytic systems and optimized reaction conditions can help achieve high yields and minimize by-products. Additionally, purification steps such as distillation and crystallization are employed to obtain the compound in its pure form .
Chemical Reactions Analysis
Types of Reactions
Acetic acid;cyclohexane-1,2-diamine undergoes various chemical reactions, including oxidation, reduction, and substitution reactions. These reactions are influenced by the functional groups present in the compound, such as the carboxylic acid group and the amine groups.
Common Reagents and Conditions
Oxidation: The compound can be oxidized using strong oxidizing agents such as potassium permanganate or chromium trioxide.
Reduction: Reduction reactions can be carried out using reducing agents like lithium aluminum hydride or sodium borohydride.
Substitution: Substitution reactions involve the replacement of one functional group with another.
Major Products Formed
The major products formed from these reactions depend on the specific reagents and conditions used. For instance, oxidation of this compound may yield carboxylic acid derivatives, while reduction can produce amine derivatives. Substitution reactions can lead to the formation of various substituted amines or amides .
Scientific Research Applications
Acetic acid;cyclohexane-1,2-diamine has a wide range of applications in scientific research:
Mechanism of Action
The mechanism of action of acetic acid;cyclohexane-1,2-diamine involves its interaction with molecular targets such as enzymes and receptors. The compound can form hydrogen bonds and coordinate with metal ions, influencing the activity of enzymes and other biological molecules. The specific pathways involved depend on the context in which the compound is used, such as in catalysis or drug development .
Comparison with Similar Compounds
Similar Compounds
Cyclohexane-1,2-diamine: A diamine with similar structural features but lacking the acetic acid component.
Acetic acid: A simple carboxylic acid without the diamine functionality.
Cyclohexane-1,2-diamine-NNN′N′-tetra-acetic acid: A related compound with additional acetic acid groups, used as a chelating agent.
Uniqueness
Acetic acid;cyclohexane-1,2-diamine is unique due to its combination of carboxylic acid and diamine functionalities, which confer distinct chemical and biological properties. This dual functionality allows the compound to participate in a wide range of reactions and applications, making it a valuable tool in various fields of research and industry.
Properties
CAS No. |
957771-42-5 |
---|---|
Molecular Formula |
C10H22N2O4 |
Molecular Weight |
234.29 g/mol |
IUPAC Name |
acetic acid;cyclohexane-1,2-diamine |
InChI |
InChI=1S/C6H14N2.2C2H4O2/c7-5-3-1-2-4-6(5)8;2*1-2(3)4/h5-6H,1-4,7-8H2;2*1H3,(H,3,4) |
InChI Key |
OGZVEPYZKPDQBT-UHFFFAOYSA-N |
Canonical SMILES |
CC(=O)O.CC(=O)O.C1CCC(C(C1)N)N |
Origin of Product |
United States |
Disclaimer and Information on In-Vitro Research Products
Please be aware that all articles and product information presented on BenchChem are intended solely for informational purposes. The products available for purchase on BenchChem are specifically designed for in-vitro studies, which are conducted outside of living organisms. In-vitro studies, derived from the Latin term "in glass," involve experiments performed in controlled laboratory settings using cells or tissues. It is important to note that these products are not categorized as medicines or drugs, and they have not received approval from the FDA for the prevention, treatment, or cure of any medical condition, ailment, or disease. We must emphasize that any form of bodily introduction of these products into humans or animals is strictly prohibited by law. It is essential to adhere to these guidelines to ensure compliance with legal and ethical standards in research and experimentation.