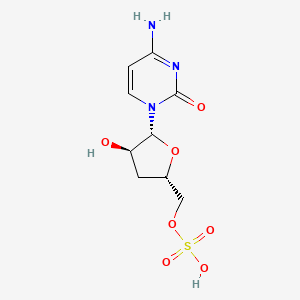
3'-Deoxycytidine sulphate
- Click on QUICK INQUIRY to receive a quote from our team of experts.
- With the quality product at a COMPETITIVE price, you can focus more on your research.
Overview
Description
This modification alters its biochemical properties, including phosphorylation efficiency and metabolic stability. While the parent compound, 3'-deoxycytidine, has been studied for antiviral activity, its sulphate derivative may enhance solubility or stability for therapeutic applications.
Preparation Methods
Synthetic Routes and Reaction Conditions
The preparation of 3’-Deoxycytidine sulphate involves the reaction of deoxycytidine with sulphuric acid. The process typically includes the following steps:
Dissolution: Deoxycytidine is dissolved in an appropriate solvent, such as methanol.
Reaction: Sulphuric acid is added to the solution, and the mixture is stirred at a controlled temperature.
Crystallization: The product is allowed to crystallize out of the solution.
Purification: The crystals are filtered, washed, and dried to obtain pure 3’-Deoxycytidine sulphate.
Industrial Production Methods
Industrial production of 3’-Deoxycytidine sulphate may involve large-scale synthesis using similar reaction conditions but optimized for higher yield and purity. This can include the use of automated reactors, precise temperature control, and advanced purification techniques to ensure consistent quality .
Chemical Reactions Analysis
Reactivity of Sulfate Radicals with Cytidine Derivatives
Sulfate radicals (SO₄·⁻) exhibit distinct reactivity patterns depending on the structure of the nucleoside. For example:
-
2'-Deoxycytidine (1a) : SO₄·⁻ primarily forms N-centered base radicals (λₘₐₓ = 400 nm) via sulfate release and deprotonation at nitrogen. Minor C-centered OH-adduct radicals are also observed but decay rapidly (second-order kinetics). Cytosine release is minimal (G = 0.14 × 10⁻⁷ mol J⁻¹) .
-
Cytidine (1b) : SO₄·⁻ generates sugar radicals (λₘₐₓ = 530–600 nm) due to intramolecular attack by the ribose 5'-OH group on the sulfate adduct. This leads to higher cytosine yields (G = 2.8–7 × 10⁻⁷ mol J⁻¹) .
For 3'-deoxycytidine , the absence of a 3'-OH group may shift reactivity toward base-centered pathways, akin to 2'-deoxycytidine, though steric and electronic effects could alter intermediate stability.
Sulfate Radical Adduct Formation
-
SO₄·⁻ likely attacks the N3 position of the cytosine base, forming a transient sulfate adduct radical. This intermediate could undergo:
Base Modification and Degradation
-
Deamination : Alkylation at N3 (e.g., by sulfate adducts) accelerates cytosine deamination. For 3-methyl-2'-deoxycytidine, deamination occurs 4,000× faster than in unmodified cytidine (t₁/₂ = 406 h vs. ~170,000 h) .
-
Deglycosylation : Sulfate adducts at N3 may weaken the glycosidic bond, leading to base release (e.g., free cytosine) .
Sugar Backbone Reactions
-
The lack of a 3'-OH group in 3'-deoxycytidine minimizes ribose-specific reactions (e.g., 5'-OH intramolecular attacks observed in cytidine) .
Key Data and Comparative Analysis
Influence of Reaction Conditions
-
pH : Acidic conditions stabilize N-centered radicals (e.g., λₘₐₓ shifts from 400 nm to 450 nm in 2'-deoxycytidine) .
-
Buffers : Phosphate buffers accelerate sulfate release from adducts, suppressing sugar radical formation .
-
Dose Rate : Chain reactions involving peroxodisulfate increase cytosine yields at low dose rates (γ-radiolysis) .
Structural and Mechanistic Insights
-
Third Mg²⁺ Site : In polymerase-associated reactions, a third Mg²⁺ ion (C-site) coordinates with nonbridging oxygens during phosphodiester bond formation . While unrelated to sulfate chemistry, this highlights the role of metal ions in stabilizing intermediates.
-
Synthetic Analogues : 3',5'-Dithio-2'-deoxycytidine derivatives (e.g., S,S'-diacetyl compounds) are synthesized via thioacetate substitution, suggesting potential routes for sulfate functionalization .
Scientific Research Applications
3’-Deoxycytidine sulphate has several applications in scientific research:
Chemistry: Used as a precursor in the synthesis of other nucleoside analogs.
Biology: Studied for its role in DNA synthesis and repair mechanisms.
Medicine: Investigated for its potential use in anticancer therapies, particularly in the treatment of myelodysplastic syndromes and acute myeloid leukemia.
Industry: Utilized in the production of pharmaceuticals and as a biomarker for certain types of cancer
Mechanism of Action
The mechanism of action of 3’-Deoxycytidine sulphate involves its incorporation into DNA, where it can interfere with DNA synthesis and repair. It is phosphorylated by deoxycytidine kinase to form deoxycytidine monophosphate, which can then be further phosphorylated to form deoxycytidine triphosphate. This triphosphate form can be incorporated into DNA, leading to chain termination and inhibition of DNA synthesis .
Comparison with Similar Compounds
Structural and Functional Differences
The following table summarizes key structural features and biological activities of 3'-deoxycytidine and related nucleoside analogs:
Key Research Findings
This inactivity is attributed to inefficient triphosphate formation or rapid metabolic degradation in cells . In contrast, ara-C and gemcitabine exhibit potent antiviral and anticancer effects due to efficient phosphorylation and incorporation into nucleic acids .
DNA Methylation Inhibition :
- Decitabine (5-aza-2'-deoxycytidine) demethylates DNA by irreversibly binding methyltransferases, restoring gene expression (e.g., PKC1 in cardiac hypertrophy). It outperformed zebularine in delaying bladder cancer cell growth (79.8% vs. 17.5% cell doubling time extension in RT4 cells) .
Metabolic Competition :
- Elevated dCTP levels in cancer cells (e.g., UMUC3GR bladder cancer) confer resistance to gemcitabine by outcompeting the drug for DNA incorporation. This highlights the interplay between nucleotide biosynthesis pathways and drug efficacy .
Enzymatic Substrate Specificity :
- 3'-Deoxycytidine is a poor substrate for deoxycytidine kinase compared to ara-C and EdC , limiting its therapeutic utility. Modifications like the ethynyl group in EdC enhance its utility in research without requiring kinase activity .
Pharmacokinetic and Stability Considerations
- Intracellular Retention: Analogues like 4'-thio-arabinofuranosylcytosine (T-araC) achieve prolonged intracellular retention of triphosphate forms, enhancing cytotoxicity despite lower kinase affinity .
- Metabolic Feedback : Deoxycytidine kinase activity is tightly regulated by dCTP levels. Inhibitors of ribonucleotide reductase (e.g., hydroxyurea) enhance salvage pathway activity by reducing dCTP-mediated feedback inhibition .
Biological Activity
3'-Deoxycytidine sulphate (3'-dC-SO₄) is a nucleotide analog that has garnered attention due to its potential applications in cancer therapy and antiviral treatments. As a derivative of deoxycytidine, its biological activity is influenced by its structural modifications, which can affect its metabolism, mechanism of action, and therapeutic efficacy. This article explores the biological activity of this compound, including its synthesis, cellular uptake, metabolic pathways, and clinical implications.
This compound can be synthesized through various chemical processes that modify the deoxyribose sugar and the cytosine base. The synthesis typically involves protecting groups to facilitate selective reactions, followed by deprotection to yield the final product. The chemical structure is critical for its biological activity as it impacts solubility, stability, and interaction with cellular enzymes.
The biological activity of this compound primarily relies on its ability to be phosphorylated into active metabolites. Similar to other nucleoside analogs like gemcitabine, 3'-dC-SO₄ requires phosphorylation by deoxycytidine kinase (DCK) to form its triphosphate form (dC-SO₄-TP), which can then incorporate into DNA during replication. This incorporation disrupts normal DNA synthesis and function, leading to cytotoxic effects in rapidly dividing cells.
Table 1: Comparison of Nucleoside Analog Mechanisms
Compound | Required Kinase | Active Form | Mechanism of Action |
---|---|---|---|
3'-Deoxycytidine | DCK | dC-SO₄-TP | Incorporation into DNA |
Gemcitabine | DCK | dFdCTP | Inhibition of ribonucleotide reductase |
Cytosine Arabinoside | DCK | Ara-CTP | Incorporation into DNA |
Biological Activity in Cancer Cells
Research indicates that this compound exhibits significant growth-inhibitory effects against various cancer cell lines. For instance, studies have shown that it can bypass resistance mechanisms associated with deoxycytidine kinase deficiency. In DCK-deficient cell lines, 3'-dC-SO₄ demonstrates enhanced cytotoxicity compared to gemcitabine, suggesting its potential as an effective treatment option for tumors resistant to conventional therapies .
Case Study: Efficacy in DCK-Deficient Tumors
In a comparative study involving DCK-deficient cell lines (AG6000 and CEM/dCK-), this compound was found to be approximately four times more effective than gemcitabine. This highlights its potential utility in treating cancers where DCK expression is compromised .
Antiviral Activity
Beyond oncology, this compound has shown promise in antiviral applications. Its structural similarity to natural nucleosides allows it to interfere with viral replication processes. Studies have demonstrated that compounds like 3',4'-didehydro-3'-deoxycytidine exhibit significant antiviral properties against SARS-CoV-2, indicating a potential role for related compounds in managing viral infections .
Pharmacokinetics and Metabolism
The pharmacokinetics of this compound are crucial for understanding its therapeutic window and dosing strategies. Following administration, the compound undergoes rapid phosphorylation within cells. The rate-limiting step is the conversion by DCK; thus, factors affecting DCK activity can significantly influence the drug's efficacy .
Table 2: Pharmacokinetic Profiles
Parameter | Value |
---|---|
Bioavailability | High |
Peak Plasma Concentration | Varies by formulation |
Half-Life | Short (< 2 hours) |
Clinical Implications
The clinical implications of using this compound are profound. Its ability to overcome resistance mechanisms in cancer therapy positions it as a candidate for combination therapies with existing chemotherapeutics. Furthermore, ongoing research into its antiviral properties may expand its applications beyond oncology.
Q & A
Basic Research Questions
Q. What synthesis protocols are recommended for producing 3'-Deoxycytidine sulphate analogs, and how do reaction conditions impact yield?
Established methods for synthesizing 2'-deoxycytidine derivatives, such as N4-dodecyl-5-methyl-2'-deoxycytidine (Ala-54) and 3'-amino analogs, involve modifying nucleoside precursors via alkylation or phosphorylation reactions . Yield optimization requires precise control of reaction temperature, stoichiometry of reagents (e.g., dodecyl groups), and purification via column chromatography. While this compound synthesis is not explicitly documented, analogous protocols for 2'-derivatives suggest sulfate groups can be introduced via sulfonation under anhydrous conditions.
Q. Which analytical techniques are critical for confirming the structural integrity of this compound?
High-performance liquid chromatography (HPLC) with UV detection is recommended for purity assessment, as demonstrated for 5-methyl-2′-deoxycytidine in methylation studies . Nuclear magnetic resonance (NMR) spectroscopy, particularly 1H- and 13C-NMR, can resolve stereochemical details at the 3' position, while mass spectrometry (MS) confirms molecular weight and fragmentation patterns, as shown for 2′-deoxycytidine diphosphate .
Q. What safety protocols should guide the handling of this compound in laboratory settings?
Based on safety data sheets (SDS) for related nucleotides, researchers should wear nitrile gloves, safety goggles, and lab coats to prevent skin/eye contact. Workstations must have adequate ventilation and accessible eyewash stations. Spills should be contained mechanically (e.g., absorbent pads) to avoid environmental contamination .
Q. How should this compound be stored to maintain stability?
Similar to deoxycytidine triphosphate, storage at -20°C in anhydrous, light-protected containers is advised. Lyophilized forms are stable for years, whereas aqueous solutions may require buffering at neutral pH to prevent hydrolysis .
Q. What in vitro models are suitable for preliminary testing of this compound’s bioactivity?
Keratinocyte scratch assays (as in ) and osteosarcoma cell lines (e.g., treated with demethylating agents like 5-aza-2'-deoxycytidine) are validated models for studying nucleotide-mediated effects on DNA repair or methylation .
Advanced Research Questions
Q. How can contradictory data on this compound’s epigenetic effects across cell lines be resolved?
Discrepancies in demethylation efficacy, as seen with 5-aza-2'-deoxycytidine in gastric vs. osteosarcoma cells , may arise from cell-specific DNA methyltransferase (DNMT) expression. Researchers should:
- Perform dose-response curves (e.g., 1×10−7 to 3×10−7 M) .
- Validate results using bisulfite sequencing and qPCR for target genes (e.g., GPX3 ).
- Include DNMT knockdown controls (e.g., DNMT3a shRNA) to confirm mechanism .
Q. What experimental designs are essential for studying this compound’s impact on DNA repair pathways?
- Use scratch-wound assays in keratinocytes to simulate DNA damage, paired with caspase-8 mRNA quantification via qPCR and protein analysis via Western blot .
- Compare results to untreated controls and validate with apoptosis markers (e.g., APAF-1 methylation via COBRA) .
- Apply two-tailed t-tests for statistical rigor, with significance thresholds at *P≤0.05 .
Q. How does the 3' sulfate group influence enzymatic incorporation into nucleic acids compared to 2'-deoxycytidine derivatives?
Structural studies using NMR (e.g., ’s analysis of oligonucleotide melting temperatures) suggest 3' modifications alter polymerase binding affinity. Researchers can:
- Conduct primer extension assays with Taq polymerase and dNTP analogs.
- Compare incorporation rates via gel electrophoresis or fluorescent labeling.
- Use X-ray crystallography to resolve 3' sulfate interactions in polymerase active sites .
Q. What computational and biophysical methods can predict this compound’s binding to DNMTs?
- Molecular docking simulations (e.g., AutoDock Vina) can model interactions between the 3' sulfate and DNMT catalytic pockets.
- Validate predictions with isothermal titration calorimetry (ITC) to measure binding thermodynamics .
- Cross-reference with methylation data from cell-based assays to confirm functional relevance .
Q. How can researchers ensure specificity when using this compound as a demethylation agent?
- Combine treatment with DNMT inhibitors (e.g., 5-aza-2'-deoxycytidine) and gene-specific methylation analysis (e.g., pyrosequencing).
- Perform genome-wide methylation arrays to identify off-target effects.
- Use clustered regularly interspaced short palindromic repeats (CRISPR) knockout models to isolate DNMT-dependent pathways .
Q. Methodological Notes
- Data Contradiction Analysis : Always include technical replicates (n≥3) and normalize results to housekeeping genes (e.g., α-Tubulin) to mitigate variability .
- Advanced Techniques : For structural studies, tandem MS/MS and high-resolution NMR (600 MHz or above) are critical for resolving 3' modification effects .
- Ethical Compliance : Follow institutional guidelines for hazardous waste disposal, particularly for sulfated compounds, to prevent environmental release .
Properties
CAS No. |
75489-87-1 |
---|---|
Molecular Formula |
C9H13N3O7S |
Molecular Weight |
307.28 g/mol |
IUPAC Name |
[(2S,4R,5R)-5-(4-amino-2-oxopyrimidin-1-yl)-4-hydroxyoxolan-2-yl]methyl hydrogen sulfate |
InChI |
InChI=1S/C9H13N3O7S/c10-7-1-2-12(9(14)11-7)8-6(13)3-5(19-8)4-18-20(15,16)17/h1-2,5-6,8,13H,3-4H2,(H2,10,11,14)(H,15,16,17)/t5-,6+,8+/m0/s1 |
InChI Key |
HUKMCHOXXHABCA-SHYZEUOFSA-N |
Isomeric SMILES |
C1[C@H](O[C@H]([C@@H]1O)N2C=CC(=NC2=O)N)COS(=O)(=O)O |
Canonical SMILES |
C1C(OC(C1O)N2C=CC(=NC2=O)N)COS(=O)(=O)O |
Origin of Product |
United States |
Disclaimer and Information on In-Vitro Research Products
Please be aware that all articles and product information presented on BenchChem are intended solely for informational purposes. The products available for purchase on BenchChem are specifically designed for in-vitro studies, which are conducted outside of living organisms. In-vitro studies, derived from the Latin term "in glass," involve experiments performed in controlled laboratory settings using cells or tissues. It is important to note that these products are not categorized as medicines or drugs, and they have not received approval from the FDA for the prevention, treatment, or cure of any medical condition, ailment, or disease. We must emphasize that any form of bodily introduction of these products into humans or animals is strictly prohibited by law. It is essential to adhere to these guidelines to ensure compliance with legal and ethical standards in research and experimentation.