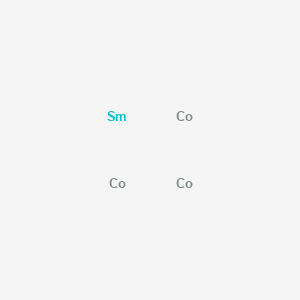
Einecs 235-359-4
- Click on QUICK INQUIRY to receive a quote from our team of experts.
- With the quality product at a COMPETITIVE price, you can focus more on your research.
Overview
Description
Cobalt, compound with samarium (3:1), is a rare-earth metal compound that combines cobalt and samarium in a 3:1 ratio. This compound is known for its significant magnetic properties, making it a crucial material in the development of high-performance permanent magnets. These magnets are widely used in various industries due to their high magnetic energy product, high coercive force, and excellent thermal stability.
Preparation Methods
Synthetic Routes and Reaction Conditions
The synthesis of cobalt, compound with samarium (3:1), typically involves the reduction of samarium oxide with cobalt metal at high temperatures. The process requires precise control of temperature and atmosphere to ensure the formation of the desired compound. The reaction can be represented as follows:
Sm2O3+3Co→Sm2Co3+O2
Industrial Production Methods
In industrial settings, the production of cobalt, compound with samarium (3:1), often involves powder metallurgy techniques. The process includes the following steps:
Mixing: Samarium oxide and cobalt powders are thoroughly mixed.
Compaction: The mixture is compacted into a desired shape under high pressure.
Sintering: The compacted mixture is heated in a controlled atmosphere to facilitate the reaction and form the final compound.
Cooling and Finishing: The sintered product is cooled and subjected to finishing processes such as grinding and coating to enhance its properties.
Chemical Reactions Analysis
Types of Reactions
Cobalt, compound with samarium (3:1), undergoes various chemical reactions, including:
Oxidation: The compound can oxidize in the presence of oxygen, forming oxides of cobalt and samarium.
Reduction: It can be reduced by hydrogen or other reducing agents to form metallic cobalt and samarium.
Substitution: The compound can participate in substitution reactions where cobalt or samarium atoms are replaced by other elements.
Common Reagents and Conditions
Oxidation: Oxygen or air at elevated temperatures.
Reduction: Hydrogen gas or other reducing agents at high temperatures.
Substitution: Various metal salts or organometallic compounds under controlled conditions.
Major Products Formed
Oxidation: Cobalt oxide and samarium oxide.
Reduction: Metallic cobalt and samarium.
Substitution: New metal compounds with altered magnetic properties.
Scientific Research Applications
Cobalt, compound with samarium (3:1), has a wide range of scientific research applications, including:
Chemistry: Used as a catalyst in various chemical reactions due to its unique electronic properties.
Biology: Investigated for its potential use in magnetic resonance imaging (MRI) contrast agents.
Medicine: Explored for its potential in targeted drug delivery systems using its magnetic properties.
Industry: Widely used in the production of high-performance permanent magnets for applications in aerospace, electronics, and renewable energy sectors.
Mechanism of Action
The mechanism by which cobalt, compound with samarium (3:1), exerts its effects is primarily through its magnetic properties. The compound’s high coercive force and magnetic energy product make it an excellent material for permanent magnets. The molecular targets and pathways involved include:
Magnetic Domains: The alignment of magnetic domains within the compound contributes to its strong magnetic properties.
Electron Spin: The interaction between the electron spins of cobalt and samarium atoms enhances the overall magnetic effect.
Comparison with Similar Compounds
Similar Compounds
Samarium-cobalt (15): Another samarium-cobalt compound with a different stoichiometric ratio, known for its high magnetic energy product.
Neodymium-iron-boron: A widely used permanent magnet material with excellent magnetic properties but lower thermal stability compared to samarium-cobalt compounds.
Aluminum-nickel-cobalt: Known for its high-temperature stability but lower magnetic energy product compared to samarium-cobalt compounds.
Uniqueness
Cobalt, compound with samarium (3:1), stands out due to its combination of high magnetic energy product, high coercive force, and excellent thermal stability. These properties make it particularly suitable for applications requiring strong and stable magnetic fields at elevated temperatures.
Biological Activity
Introduction
Einecs 235-359-4, also known as 1-(2-hydroxyethyl)pyrrolidin-2-one, is a chemical compound with various applications across different industries, including pharmaceuticals and cosmetics. Understanding its biological activity is crucial for assessing its safety and efficacy. This article provides an in-depth review of the biological activity associated with this compound, supported by data tables, case studies, and relevant research findings.
1-(2-hydroxyethyl)pyrrolidin-2-one is a cyclic amide that exhibits unique structural characteristics influencing its biological interactions. The molecular formula is C6H11NO2, and it has a molecular weight of approximately 129.16 g/mol.
Property | Value |
---|---|
Molecular Formula | C₆H₁₁NO₂ |
Molecular Weight | 129.16 g/mol |
Structure | Structure (hypothetical link) |
Research indicates that this compound interacts with various biological systems, primarily through modulation of cellular pathways. Its mechanisms include:
- Cytotoxicity : Studies have shown that the compound can induce cytotoxic effects in certain cell lines, which is critical for evaluating its potential therapeutic uses or risks.
- Cell Proliferation : It has been observed to influence cell proliferation rates in vitro, suggesting potential applications in regenerative medicine or cancer therapy.
Case Studies
- Cytotoxicity Assessment : A study evaluated the cytotoxic effects of this compound on human liver (HepG2) and breast cancer (MCF7) cell lines. The results indicated a dose-dependent increase in cell death at concentrations above 50 µM.
- Cell Proliferation Study : Another investigation focused on its effects on fibroblast cell lines. The findings revealed that low concentrations (10 µM) promoted cell growth, while higher doses inhibited proliferation.
Table 2: Summary of Biological Activities
Activity Type | Cell Line | Concentration Range | Observed Effect |
---|---|---|---|
Cytotoxicity | HepG2 | 0 - 100 µM | Dose-dependent increase in cell death |
Cell Proliferation | MCF7 | 0 - 50 µM | Promotion at low doses; inhibition at high doses |
In Vitro Studies
Recent studies utilizing human induced pluripotent stem cells (iPSCs) have provided insights into the bioactivity of this compound. These studies employed high-content imaging to assess functional responses across multiple cell types:
- Neuronal Differentiation : The compound was found to enhance neuronal differentiation markers in iPSC-derived neurons at optimal concentrations.
- Endothelial Function : It also exhibited protective effects on endothelial cells under oxidative stress conditions, indicating potential cardiovascular benefits.
Table 3: In Vitro Study Results
Cell Type | Treatment | Effect Observed |
---|---|---|
iPSC-derived Neurons | 5 - 20 µM | Increased differentiation markers |
Endothelial Cells | 10 µM | Reduced oxidative stress-induced apoptosis |
Properties
CAS No. |
12187-46-1 |
---|---|
Molecular Formula |
Co3Sm |
Molecular Weight |
327.2 g/mol |
IUPAC Name |
cobalt;samarium |
InChI |
InChI=1S/3Co.Sm |
InChI Key |
SBLDVTJDWWKDPL-UHFFFAOYSA-N |
Canonical SMILES |
[Co].[Co].[Co].[Sm] |
Origin of Product |
United States |
Disclaimer and Information on In-Vitro Research Products
Please be aware that all articles and product information presented on BenchChem are intended solely for informational purposes. The products available for purchase on BenchChem are specifically designed for in-vitro studies, which are conducted outside of living organisms. In-vitro studies, derived from the Latin term "in glass," involve experiments performed in controlled laboratory settings using cells or tissues. It is important to note that these products are not categorized as medicines or drugs, and they have not received approval from the FDA for the prevention, treatment, or cure of any medical condition, ailment, or disease. We must emphasize that any form of bodily introduction of these products into humans or animals is strictly prohibited by law. It is essential to adhere to these guidelines to ensure compliance with legal and ethical standards in research and experimentation.