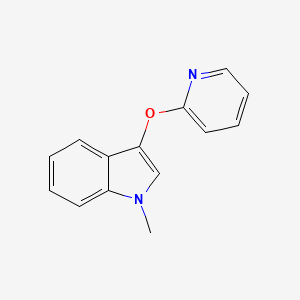
1-Methyl-3-(pyridin-2-yloxy)-1H-indole
- Click on QUICK INQUIRY to receive a quote from our team of experts.
- With the quality product at a COMPETITIVE price, you can focus more on your research.
Overview
Description
1-Methyl-3-(pyridin-2-yloxy)-1H-indole is a heterocyclic compound that features an indole core substituted with a methyl group at the nitrogen atom and a pyridin-2-yloxy group at the third position. This compound is of significant interest in medicinal chemistry due to its potential biological activities and its role as a building block in the synthesis of more complex molecules.
Preparation Methods
Synthetic Routes and Reaction Conditions: The synthesis of 1-Methyl-3-(pyridin-2-yloxy)-1H-indole typically involves the reaction of 1-methylindole with a pyridin-2-yloxy halide under basic conditions. A common method includes the use of a base such as potassium carbonate in a polar aprotic solvent like dimethylformamide (DMF). The reaction is carried out at elevated temperatures to facilitate the nucleophilic substitution reaction.
Industrial Production Methods: Industrial production of this compound may involve similar synthetic routes but optimized for large-scale production. This includes the use of continuous flow reactors to ensure consistent reaction conditions and high yields. The choice of solvents and reagents is also optimized to minimize costs and environmental impact.
Chemical Reactions Analysis
Types of Reactions: 1-Methyl-3-(pyridin-2-yloxy)-1H-indole can undergo various chemical reactions, including:
Oxidation: The indole ring can be oxidized using reagents like potassium permanganate or chromium trioxide.
Reduction: The compound can be reduced using hydrogenation catalysts such as palladium on carbon.
Substitution: The pyridin-2-yloxy group can be substituted with other nucleophiles under appropriate conditions.
Common Reagents and Conditions:
Oxidation: Potassium permanganate in an acidic medium.
Reduction: Hydrogen gas with palladium on carbon catalyst.
Substitution: Sodium hydride in DMF for nucleophilic substitution reactions.
Major Products Formed:
Oxidation: Oxidized derivatives of the indole ring.
Reduction: Reduced forms of the indole ring.
Substitution: Various substituted derivatives depending on the nucleophile used.
Scientific Research Applications
1-Methyl-3-(pyridin-2-yloxy)-1H-indole has several applications in scientific research:
Chemistry: Used as a building block for the synthesis of more complex heterocyclic compounds.
Biology: Investigated for its potential biological activities, including antimicrobial and anticancer properties.
Medicine: Explored as a potential lead compound for drug development due to its ability to interact with various biological targets.
Industry: Utilized in the synthesis of dyes, pigments, and other industrial chemicals.
Mechanism of Action
The mechanism of action of 1-Methyl-3-(pyridin-2-yloxy)-1H-indole involves its interaction with specific molecular targets. The compound can bind to enzymes or receptors, altering their activity. For example, it may inhibit certain enzymes involved in cell proliferation, leading to potential anticancer effects. The exact pathways and targets depend on the specific biological context and the modifications made to the compound.
Comparison with Similar Compounds
1-Methylindole: Lacks the pyridin-2-yloxy group, making it less versatile in terms of chemical reactivity.
3-(Pyridin-2-yloxy)indole: Similar structure but without the methyl group at the nitrogen atom.
2-Methyl-3-(pyridin-2-yloxy)indole: A positional isomer with different biological and chemical properties.
Uniqueness: 1-Methyl-3-(pyridin-2-yloxy)-1H-indole is unique due to the presence of both the methyl group and the pyridin-2-yloxy group, which confer distinct chemical reactivity and biological activity. This combination allows for a wide range of modifications and applications in various fields of research.
Q & A
Q. Basic: What are the optimal synthetic routes for 1-Methyl-3-(pyridin-2-yloxy)-1H-indole?
Methodological Answer:
The synthesis of this compound can be optimized using iodine catalysis or transition-metal-mediated borylation. For example:
- Iodine-catalyzed electrophilic substitution : Under optimized conditions (10 mol% I₂ in MeCN at 40°C for 5 hours), yields up to 98% can be achieved for analogous indole derivatives .
- Borylation strategies : Using a general procedure for indole borylation, 1-methyl-1H-indole derivatives can be functionalized with a dioxaborolane group in 70% yield via iridium-catalyzed C–H activation .
Q. Advanced: How can researchers resolve contradictions in reaction yields when varying catalysts or conditions?
Methodological Answer:
Contradictions in yields often arise from temperature, catalyst loading, or solvent effects. Systematic optimization is critical:
- Case Study : In iodine-catalyzed reactions, increasing temperature from room temperature (rt) to 40°C improved yields from 51% to 98% for similar substrates, highlighting the importance of thermal activation .
- Statistical Analysis : Use Design of Experiments (DoE) to evaluate interactions between variables (e.g., catalyst concentration, solvent polarity). Reference yield data from analogous reactions (e.g., FeCl₃ vs. I₂ catalysis) to identify outliers .
Q. Basic: How is the crystal structure of this compound determined?
Methodological Answer:
X-ray crystallography is the gold standard:
- Data Collection : Use SHELX programs (e.g., SHELXL for refinement) to process diffraction data. For example, the dihedral angle between indole and pyridine rings in analogous compounds was reported as 52.13°, with intramolecular C–H···F contacts .
- Visualization : ORTEP-III with a GUI generates thermal ellipsoid plots to visualize molecular geometry and intermolecular interactions .
Q. Advanced: What computational methods are used to predict biological interactions of this compound?
Methodological Answer:
- Molecular Docking : Perform docking studies (e.g., AutoDock Vina) to predict binding affinities to targets like enzymes or receptors. For indole derivatives, prioritize π-π stacking and hydrogen-bonding interactions observed in similar structures .
- MD Simulations : Use GROMACS or AMBER to simulate dynamic behavior in solvated systems, focusing on conformational stability over nanosecond timescales .
Q. Basic: What spectroscopic techniques are used for characterization?
Methodological Answer:
- NMR/IR Spectroscopy :
- Mass Spectrometry : High-resolution MS (HRMS) confirms molecular weight (e.g., exact mass 264.1001 for C₁₅H₁₂N₂O) .
Q. Advanced: How to evaluate the biological activity of this compound in antimicrobial assays?
Methodological Answer:
- Biofilm Disruption Assays :
- Dose-Response Analysis : Calculate IC₅₀ values using nonlinear regression models (e.g., GraphPad Prism) .
Q. Advanced: How can functionalization via borylation enhance reactivity for further applications?
Methodological Answer:
- Suzuki-Miyaura Coupling : Introduce boronate groups (e.g., 1-methyl-3-(dioxaborolanyl)-1H-indole) to enable cross-coupling with aryl halides, expanding access to biaryl structures .
- Mechanistic Insight : Monitor reaction progress via ¹¹B NMR to confirm boron incorporation and optimize catalyst systems (e.g., Ir/phosphine ligands) for regioselectivity .
Q. Basic: What are the key intramolecular interactions affecting conformational stability?
Methodological Answer:
- Short Contacts : Analyze X-ray data for C–H···X (X = O, N, F) interactions. For example, three intramolecular C–H···F contacts (<2.5 Å) were observed in a fluorinated analog, stabilizing the folded conformation .
- Torsional Angles : Use Mercury Software to calculate dihedral angles between aromatic rings, which influence π-conjugation and solubility .
Q. Advanced: How to troubleshoot low yields in large-scale synthesis?
Methodological Answer:
- Purification Challenges : Employ flash chromatography with gradients (e.g., hexane/EtOAc) to separate regioisomers. For polar byproducts, use preparative HPLC .
- Scale-Up Adjustments : Reduce catalyst loading (e.g., from 10 mol% to 5 mol% I₂) and switch to cost-effective solvents (e.g., toluene instead of MeCN) while monitoring reaction kinetics .
Q. Basic: What are the safety and handling protocols for this compound?
Methodological Answer:
Properties
Molecular Formula |
C14H12N2O |
---|---|
Molecular Weight |
224.26 g/mol |
IUPAC Name |
1-methyl-3-pyridin-2-yloxyindole |
InChI |
InChI=1S/C14H12N2O/c1-16-10-13(11-6-2-3-7-12(11)16)17-14-8-4-5-9-15-14/h2-10H,1H3 |
InChI Key |
BOJLLRRSENCQDY-UHFFFAOYSA-N |
Canonical SMILES |
CN1C=C(C2=CC=CC=C21)OC3=CC=CC=N3 |
Origin of Product |
United States |
Disclaimer and Information on In-Vitro Research Products
Please be aware that all articles and product information presented on BenchChem are intended solely for informational purposes. The products available for purchase on BenchChem are specifically designed for in-vitro studies, which are conducted outside of living organisms. In-vitro studies, derived from the Latin term "in glass," involve experiments performed in controlled laboratory settings using cells or tissues. It is important to note that these products are not categorized as medicines or drugs, and they have not received approval from the FDA for the prevention, treatment, or cure of any medical condition, ailment, or disease. We must emphasize that any form of bodily introduction of these products into humans or animals is strictly prohibited by law. It is essential to adhere to these guidelines to ensure compliance with legal and ethical standards in research and experimentation.