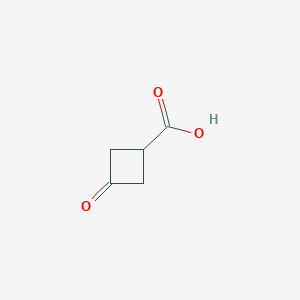
3-Oxocyclobutanecarboxylic acid
Overview
Description
3-Oxocyclobutanecarboxylic acid (CAS 23761-23-1) is a strained cyclic ketocarboxylic acid with the molecular formula C₅H₆O₃ and a molecular weight of 114.10 g/mol . It features a cyclobutane ring substituted with a ketone and a carboxylic acid group, making it the smallest known cyclic ketocarboxylic acid . The compound crystallizes in a monoclinic system (space group P2₁/c) with unit cell parameters a = 8.8858 Å, b = 5.3631 Å, c = 11.625 Å, and β = 106.899° . Its non-planar cyclobutane ring exhibits a shallow fold (14.3° dihedral angle) and significant ring strain, reflected in internal angles averaging 89.6° .
The compound’s infrared spectrum shows distinct carbonyl peaks at 1786 cm⁻¹ (ketone) and 1696 cm⁻¹ (carboxylic acid dimer) in the solid state . In solution, dimerization persists, with peaks shifting to 1797 cm⁻¹ and 1709 cm⁻¹ . Safety data classify it as hazardous (H315, H318, H302, H335), requiring precautions against skin/eye contact and inhalation .
Preparation Methods
Cyclocondensation and Hydrolysis Route
Reaction Mechanism and Procedure
The method described in CN105037130A employs a two-step cyclocondensation and hydrolysis strategy . In the first step, diisopropyl malonate reacts with 2,2-dimethoxy-1,3-dibromopropane in dimethylformamide (DMF) at 140°C for 96 hours, catalyzed by potassium tert-butoxide. This forms a cyclobutane intermediate (Product A) through nucleophilic substitution and cyclization. The second step involves hydrolyzing Product A with concentrated hydrochloric acid under reflux (102–106°C) for 120 hours, followed by dichloromethane extraction and recrystallization.
Key Parameters
-
Step 1 :
-
Molar ratio: Diisopropyl malonate : 2,2-dimethoxy-1,3-dibromopropane = 1 : 1.2
-
Temperature: 140°C (±5°C)
-
Reaction time: 96 hours
-
Solvent recovery: 50% DMF distilled post-reaction
-
-
Step 2 :
Advantages and Limitations
This route uses cost-effective reagents and avoids toxic catalysts like osmium tetroxide. However, the extended reaction time (216 hours total) and moderate yield limit its industrial viability. The use of DMF, classified as a reprotoxic solvent, necessitates stringent safety protocols .
Three-Step Synthesis from Acetone and Malononitrile
Synthetic Pathway
CN103232340A outlines a sequential approach starting with acetone bromination to form 1,3-dibromoacetone, followed by cyclization with malononitrile, and final hydrolysis .
Bromination of Acetone
Acetone reacts with bromine (1:2 molar ratio) in ethanol at 25°C for 12–16 hours, yielding 1,3-dibromoacetone. Excess bromine and hydrogen bromide are removed via distillation.
Cyclization to 3,3-Dicyanocyclobutanone
Malononitrile and 1,3-dibromoacetone undergo cyclization in DMF at 60–90°C for 24 hours, catalyzed by sodium iodide and tetrabutylammonium bromide (TBAB). The product is extracted with toluene, yielding 68–72% intermediate.
Hydrolysis to Target Compound
The nitrile groups are hydrolyzed using 50% aqueous ethanol and NaOH (70°C, 12 hours), followed by acidification (pH 3) and ethyl acetate extraction.
Performance Metrics
-
Overall yield : 52–68%
-
Purity : 99–99.2% (GC-MS)
-
Solvent recovery : Ethanol, DMF, and toluene recycled 3–5 times without yield loss .
Ozonolysis-Mediated Synthesis
Multi-Step Approach
CN103467270A utilizes a six-step sequence involving Wittig reaction intermediates and ozonolysis .
Key Steps
-
Methyl triphenylphosphine iodide synthesis : Triphenylphosphine reacts with methyl iodide in tetrahydrofuran (THF).
-
3-Benzalcyclobutanol formation : Epichlorohydrin and benzaldehyde undergo Wittig reaction at −50°C.
-
Ozonolysis : Ozone cleaves the benzal group at −50°C, followed by reductive workup to yield the carboxylic acid.
Critical Data
-
Intermediate yields :
-
3-Benzalcyclobutanol: 78%
-
3-Benzalcyclobutanenitrile: 85%
-
Final hydrolysis yield: 90%
-
-
Ozonolysis conditions :
-
Temperature: −50°C
-
Ozone flow rate: 0.5 L/min
-
Reaction monitoring: Colorimetric (blue → colorless)
-
Practical Considerations
While ozonolysis achieves high functional group selectivity, the requirement for cryogenic conditions (−50°C) and ozone generators increases operational complexity. This method is preferred for small-scale synthesis (<10 kg) in specialized laboratories .
Comparative Analysis of Synthetic Routes
Reaction Efficiency and Cost
Parameter | Method 1 | Method 2 | Method 3 |
---|---|---|---|
Total steps | 2 | 3 | 6 |
Typical yield | 16% | 60% | 45% |
Catalyst cost | Low | Moderate | High |
Solvent toxicity | High (DMF) | Moderate | Low |
Industrial Applicability
Method 2 demonstrates the best balance between yield (60%) and scalability, though it requires bromine handling infrastructure. Method 1’s low yield makes it impractical for large-scale production despite simpler chemistry. Method 3’s reliance on ozone limits its adoption to facilities with advanced safety systems .
Purity and Byproduct Profile
Chemical Reactions Analysis
3-Oxocyclobutanecarboxylic acid undergoes various chemical reactions, including oxidation, reduction, and substitution. Common reagents used in these reactions include sodium hypochlorite, acetic acid, and hydrochloric acid . For example, the oxidation of 3-hydroxycyclobutane-1,1-dicarboxylic acid potassium salt with sodium hypochlorite yields 3-oxocyclobutane-1,1-dicarboxylic acid . The major products formed from these reactions depend on the specific conditions and reagents used.
Scientific Research Applications
Pharmaceutical Applications
2.1 Drug Development
3-Oxocyclobutanecarboxylic acid is utilized in synthesizing several pharmaceutical compounds. Notably, it is a precursor for Abrocitinib, a drug approved for treating atopic dermatitis. This compound exemplifies how intermediates can lead to effective treatments for chronic conditions .
2.2 Other Therapeutic Uses
The compound is also involved in the synthesis of other significant drugs, including:
- ACK1 antibody
- MDM2 antagonist
- JAK inhibitors
- CETP inhibitors
- Kinase inhibitors
- PDE10 inhibitors
- Thrombin inhibitors
These drugs are employed in treating various conditions such as autoimmune diseases and cancers, highlighting the compound's versatility in therapeutic applications .
Organic Synthesis Applications
3.1 Synthetic Intermediates
In organic chemistry, this compound is a vital intermediate for synthesizing a wide range of compounds. Its ability to undergo transformations makes it essential in creating complex molecular structures necessary for drug discovery and development .
3.2 Organic Light Emitting Diodes (OLEDs)
Another application of this compound is in the production of organic light-emitting diodes (OLEDs). The compound's properties facilitate its use in electronic applications, showcasing its versatility beyond pharmaceuticals .
Case Studies
5.1 Case Study: Abrocitinib Development
Abrocitinib was developed using this compound as a key intermediate. The drug has shown efficacy in clinical trials for atopic dermatitis, demonstrating the compound's significance in real-world applications .
5.2 Case Study: Synthesis Optimization
Research has focused on optimizing the synthesis of this compound to enhance yield and reduce costs. New methods have been developed that avoid highly toxic reagents while maintaining efficiency and safety standards .
Mechanism of Action
The mechanism of action of 3-Oxocyclobutanecarboxylic acid involves its interaction with specific molecular targets and pathways. For instance, it is used in the synthesis of kinase inhibitors, which target specific kinases involved in cell signaling pathways. These inhibitors can modulate the activity of kinases, leading to therapeutic effects in conditions such as cancer and inflammatory diseases .
Comparison with Similar Compounds
Structural and Physical Properties
*Estimated values based on structural analogs.
Key Observations:
Ring Strain : The cyclobutane ring in this compound introduces greater angular strain (average internal angle ~89.6°) compared to larger rings like cyclopentane (~108°) or cyclohexane (~109.5°). This strain increases reactivity and influences crystal packing .
Hydrogen Bonding: Unlike 3-oxocyclopentanecarboxylic acid, which forms acid-to-ketone dimers, the cyclobutane derivative primarily adopts carboxyl dimer pairs due to steric constraints . Non-cyclic analogs like ethyl 3-oxobutanoate lack such directional interactions .
Polarity : The oxo group in this compound enhances polarity (XLogP₃ = -0.8) compared to alkyl-substituted analogs (e.g., 3,3-dimethylcyclobutanecarboxylic acid, XLogP₃ ~1.2), affecting solubility and partitioning .
Crystallographic and Spectroscopic Differences
- Crystal Packing : The cyclobutane derivative forms chiral conformations with carboxyl dimers linked by screw-axis symmetry, while larger rings (e.g., cyclohexane) adopt less strained, more symmetric packing .
- IR Signatures : The ketone stretch in this compound (1786 cm⁻¹) is higher than in 3-oxocyclopentanecarboxylic acid (~1750 cm⁻¹), reflecting increased ring strain and bond distortion .
Biological Activity
3-Oxocyclobutanecarboxylic acid (OCBCA) is a compound recognized for its significant role as a pharmaceutical intermediate. Its structural characteristics and biological activities make it valuable in the synthesis of various therapeutic agents, particularly in oncology and immunology.
This compound has a molecular formula of CHO and a molecular weight of approximately 114.10 g/mol. It is characterized by a cyclobutane ring with a ketone and carboxylic acid functional groups, which contribute to its reactivity and biological properties.
Synthesis
The synthesis of OCBCA typically involves multi-step reactions using readily available precursors. For instance, one method employs acetone, bromine, and malononitrile as starting materials, utilizing sodium iodide as an activating agent and tetrabutylammonium bromide as a phase transfer catalyst. The yield of this synthesis ranges from 52% to 68%, with a purity exceeding 99% .
Antitumor Properties
Research indicates that OCBCA serves as an intermediate in the synthesis of various antitumor agents. Its derivatives have been shown to inhibit key enzymes involved in cancer cell proliferation. For example, compounds synthesized from OCBCA have demonstrated activity against MDM2 antagonists and kinase inhibitors, crucial for targeting cancer pathways .
Immunomodulatory Effects
OCBCA has been implicated in the modulation of immune responses. Its role in the synthesis of drugs targeting autoimmune diseases suggests that it may influence inflammatory pathways, although specific mechanisms remain under investigation . The compound's ability to interfere with cellular signaling pathways makes it a candidate for further research in immunotherapy.
Inhibition of Metabolic Enzymes
Studies have also explored the potential of OCBCA in inhibiting metabolic enzymes such as ornithine aminotransferase. This inhibition can disrupt glutamine and proline metabolism in hepatocellular carcinoma, potentially depriving tumors of essential nutrients necessary for growth .
Case Studies
Case Study 1: Synthesis and Evaluation of Antitumor Agents
A study synthesized various derivatives from OCBCA to evaluate their antitumor efficacy. The derivatives were tested against different cancer cell lines, revealing IC50 values in the low micromolar range, indicating potent activity against tumor cells. These findings support the development of OCBCA-based drugs for cancer treatment.
Case Study 2: Immunomodulatory Activity Assessment
In another study, researchers assessed the immunomodulatory effects of OCBCA derivatives on T-cell activation. The results showed that certain derivatives enhanced T-cell proliferation while reducing pro-inflammatory cytokine production, suggesting a dual role in enhancing immune response while mitigating excessive inflammation.
Data Tables
Property | Value |
---|---|
Molecular Formula | CHO |
Molecular Weight | 114.10 g/mol |
Purity | 99%+ |
Yield (Synthesis Method) | 52-68% |
Antitumor Activity (IC50) | Low micromolar range |
Biological Activity | Description |
---|---|
Antitumor | Inhibits cancer cell proliferation |
Immunomodulatory | Modulates immune responses |
Enzyme Inhibition | Disrupts metabolic pathways |
Q & A
Basic Research Questions
Q. What are the key physicochemical properties of 3-oxocyclobutanecarboxylic acid, and how are they experimentally determined?
Answer: The compound (C₅H₆O₃, MW 114.10) is a white crystalline solid with a melting point of 69–70°C . Key characterization methods include:
- X-ray crystallography to resolve its non-planar cyclobutane ring (average internal angle: 89.63°) and folded conformation (dihedral angle: 14.3° between ketone and carboxyl planes) .
- Spectroscopic techniques (e.g., NMR, IR) to confirm functional groups and hydrogen bonding patterns.
- Thermogravimetric analysis to assess stability under storage conditions (recommended: sealed, dark, room temperature) .
Q. What safety protocols are critical when handling this compound in the laboratory?
Answer: The compound poses hazards including skin/eye irritation (H315, H318), respiratory toxicity (H335), and acute oral toxicity (H302) . Mitigation strategies include:
- Personal protective equipment (PPE): Gloves, goggles, and lab coats.
- Ventilation: Use fume hoods to avoid inhalation of aerosols.
- Emergency response: Immediate rinsing with water for eye/skin contact and medical consultation for ingestion .
Q. How is this compound synthesized and purified for research use?
Answer: While synthesis routes are not explicitly detailed in the evidence, purification typically involves:
- Recrystallization from polar aprotic solvents to achieve >95% purity .
- Chromatographic techniques (e.g., HPLC) for isolating stereoisomers or removing byproducts.
- Analytical validation via melting point analysis and LC-MS to confirm purity .
Advanced Research Questions
Q. How does the non-planar cyclobutane ring influence the compound’s reactivity and hydrogen-bonding behavior?
Answer: The ring’s folded conformation (dihedral angle: 14.3°) reduces eclipsing strain but introduces torsional asymmetry (O2–C5–C1–C4 torsion: 78.7°), creating a chiral environment despite the molecule’s nominal symmetry . This distortion:
- Modifies hydrogen-bonding modes: Favors carboxyl-to-ketone dimers over catemers due to steric constraints .
- Impacts reactivity: The strained ring may enhance susceptibility to nucleophilic attack at the ketone or carboxyl groups.
Q. What insights do crystal packing interactions provide for designing derivatives with tailored aggregation properties?
Answer: X-ray studies reveal two distinct dimeric motifs linked by O–H···O hydrogen bonds (2.58–2.67 Å) and C–H···O interactions . These motifs suggest:
- Steric control: Substituents on the cyclobutane ring could stabilize specific dimerization patterns (e.g., catemers vs. dimers).
- Solvent effects: Polar solvents may disrupt intermolecular H-bonds, favoring monomeric forms for reactions.
Q. How is this compound utilized as a building block in medicinal chemistry?
Answer: The compound serves as a precursor in synthesizing inhibitors targeting the von Hippel-Lindau (VHL) E3 ubiquitin ligase. For example:
- VHL inhibitor synthesis: Coupling with pyrrolidine-carboxamide derivatives yields potent cell-active compounds (54% yield via amidation) .
- Rational design: The ketone and carboxyl groups enable functionalization (e.g., amidation, esterification) for structure-activity relationship (SAR) studies.
Q. What discrepancies exist between computational predictions and experimental structural data for this compound?
Answer: Theoretical models often assume planar cyclobutane rings, but experimental data show significant folding (dihedral angle: 14.3°) and bond-length variations (e.g., C=O bonds: 1.21 Å vs. 1.23 Å in simulations) . These discrepancies highlight:
- Limitations of force fields: Current molecular mechanics models underestimate ring strain and torsional effects.
- Need for hybrid methods: Combining DFT calculations with crystallographic data improves conformational predictions.
Q. How can systematic reviews (e.g., Cochrane guidelines) be applied to resolve contradictions in hydrogen-bonding studies of γ-keto acids?
Answer: Adopting Cochrane systematic review principles :
- Literature screening: Identify all crystallographic studies of γ-keto acids (e.g., pyruvic acid, cyclopentane derivatives) .
- Data extraction: Tabulate hydrogen-bonding modes (e.g., dimers, catemers) and contextual factors (e.g., ring size, substituents).
- Meta-analysis: Statistically evaluate trends (e.g., prevalence of carboxyl-to-ketone dimers in small rings) to resolve contradictions .
Q. Methodological Notes
Properties
IUPAC Name |
3-oxocyclobutane-1-carboxylic acid | |
---|---|---|
Source | PubChem | |
URL | https://pubchem.ncbi.nlm.nih.gov | |
Description | Data deposited in or computed by PubChem | |
InChI |
InChI=1S/C5H6O3/c6-4-1-3(2-4)5(7)8/h3H,1-2H2,(H,7,8) | |
Source | PubChem | |
URL | https://pubchem.ncbi.nlm.nih.gov | |
Description | Data deposited in or computed by PubChem | |
InChI Key |
IENOFRJPUPTEMI-UHFFFAOYSA-N | |
Source | PubChem | |
URL | https://pubchem.ncbi.nlm.nih.gov | |
Description | Data deposited in or computed by PubChem | |
Canonical SMILES |
C1C(CC1=O)C(=O)O | |
Source | PubChem | |
URL | https://pubchem.ncbi.nlm.nih.gov | |
Description | Data deposited in or computed by PubChem | |
Molecular Formula |
C5H6O3 | |
Source | PubChem | |
URL | https://pubchem.ncbi.nlm.nih.gov | |
Description | Data deposited in or computed by PubChem | |
DSSTOX Substance ID |
DTXSID20406767 | |
Record name | 3-oxocyclobutanecarboxylic acid | |
Source | EPA DSSTox | |
URL | https://comptox.epa.gov/dashboard/DTXSID20406767 | |
Description | DSSTox provides a high quality public chemistry resource for supporting improved predictive toxicology. | |
Molecular Weight |
114.10 g/mol | |
Source | PubChem | |
URL | https://pubchem.ncbi.nlm.nih.gov | |
Description | Data deposited in or computed by PubChem | |
CAS No. |
23761-23-1 | |
Record name | 3-oxocyclobutanecarboxylic acid | |
Source | EPA DSSTox | |
URL | https://comptox.epa.gov/dashboard/DTXSID20406767 | |
Description | DSSTox provides a high quality public chemistry resource for supporting improved predictive toxicology. | |
Record name | 3-oxocyclobutanecarboxylic acid | |
Source | European Chemicals Agency (ECHA) | |
URL | https://echa.europa.eu/substance-information/-/substanceinfo/100.167.068 | |
Description | The European Chemicals Agency (ECHA) is an agency of the European Union which is the driving force among regulatory authorities in implementing the EU's groundbreaking chemicals legislation for the benefit of human health and the environment as well as for innovation and competitiveness. | |
Explanation | Use of the information, documents and data from the ECHA website is subject to the terms and conditions of this Legal Notice, and subject to other binding limitations provided for under applicable law, the information, documents and data made available on the ECHA website may be reproduced, distributed and/or used, totally or in part, for non-commercial purposes provided that ECHA is acknowledged as the source: "Source: European Chemicals Agency, http://echa.europa.eu/". Such acknowledgement must be included in each copy of the material. ECHA permits and encourages organisations and individuals to create links to the ECHA website under the following cumulative conditions: Links can only be made to webpages that provide a link to the Legal Notice page. | |
Retrosynthesis Analysis
AI-Powered Synthesis Planning: Our tool employs the Template_relevance Pistachio, Template_relevance Bkms_metabolic, Template_relevance Pistachio_ringbreaker, Template_relevance Reaxys, Template_relevance Reaxys_biocatalysis model, leveraging a vast database of chemical reactions to predict feasible synthetic routes.
One-Step Synthesis Focus: Specifically designed for one-step synthesis, it provides concise and direct routes for your target compounds, streamlining the synthesis process.
Accurate Predictions: Utilizing the extensive PISTACHIO, BKMS_METABOLIC, PISTACHIO_RINGBREAKER, REAXYS, REAXYS_BIOCATALYSIS database, our tool offers high-accuracy predictions, reflecting the latest in chemical research and data.
Strategy Settings
Precursor scoring | Relevance Heuristic |
---|---|
Min. plausibility | 0.01 |
Model | Template_relevance |
Template Set | Pistachio/Bkms_metabolic/Pistachio_ringbreaker/Reaxys/Reaxys_biocatalysis |
Top-N result to add to graph | 6 |
Feasible Synthetic Routes
Disclaimer and Information on In-Vitro Research Products
Please be aware that all articles and product information presented on BenchChem are intended solely for informational purposes. The products available for purchase on BenchChem are specifically designed for in-vitro studies, which are conducted outside of living organisms. In-vitro studies, derived from the Latin term "in glass," involve experiments performed in controlled laboratory settings using cells or tissues. It is important to note that these products are not categorized as medicines or drugs, and they have not received approval from the FDA for the prevention, treatment, or cure of any medical condition, ailment, or disease. We must emphasize that any form of bodily introduction of these products into humans or animals is strictly prohibited by law. It is essential to adhere to these guidelines to ensure compliance with legal and ethical standards in research and experimentation.