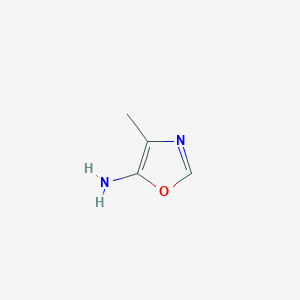
4-Methyloxazol-5-amine
Overview
Description
4-Methyloxazol-5-amine is a heterocyclic organic compound with the molecular formula C₄H₆N₂O, consisting of an oxazole ring substituted with a methyl group at position 4 and an amine group at position 5 (Figure 1). This compound is of interest due to its role as a synthetic intermediate in medicinal chemistry, particularly in the development of enzyme inhibitors and anticonvulsant agents . Its molecular weight is 98.10 g/mol, and it is typically synthesized via palladium-catalyzed cross-coupling reactions or Staudinger/aza-Wittig methodologies . Key spectral data (e.g., NMR, MS) confirm its structure, though detailed physical properties (e.g., solubility, melting point) are less documented in publicly available literature.
Preparation Methods
Direct Heating and Condensation Methods
One classical approach involves heating precursors containing oxazole rings or related heterocycles with suitable reagents to induce ring transformations and amination.
- A patent describes heating 4-methyloxazole with other compounds such as j5-phenylsulfonyl-2,5-dihydrofuran or cis-2-methylsulfonyl-1,4-dimethoxy-but-2-ene at elevated temperatures (140–150 °C) for several hours (3–8 h) to facilitate reactions leading to substituted pyridines or related compounds. Although this patent primarily focuses on pyridine derivatives, it demonstrates that 4-methyloxazole can be thermally stable and reactive under such conditions, which could be adapted for amination at position 5 by appropriate nucleophilic substitution or ring-opening/reclosing strategies.
Oxazolone Ring Synthesis and Functionalization
A significant body of literature focuses on synthesizing oxazolones (oxazole-2-ones) as intermediates, which can be subsequently modified to yield 4-methyloxazol-5-amine.
General Oxazolone Synthesis: Oxazolones are commonly synthesized by cyclization of amino acids (e.g., glycine derivatives) with aldehydes or ketones in the presence of dehydrating agents like acetic anhydride or catalysts such as zinc oxide. For example, 4-(4-hydroxybenzylidene)-2-substituted oxazol-5-ones can be prepared by reacting glycine with benzaldehyde derivatives under mild conditions.
Catalytic Methods: Microwave irradiation and catalysts like dodecatungstophosphoric acid, samarium, and ruthenium(III) chloride have been employed to accelerate the formation of 2-phenyl-5(4H)-oxazolones from hippuric acid and aldehydes or ketones, achieving high yields in short times.
Functional Group Introduction: The amino group at position 5 can be introduced via oxidation or substitution reactions on oxazolone intermediates. For instance, oxidation of purine derivatives leads to oxazolone formation with amino substituents at specific ring positions, suggesting that controlled oxidation or amination of oxazolone rings could be a pathway to this compound.
Palladium-Catalyzed Coupling Reactions
Recent synthetic strategies utilize palladium-catalyzed cross-coupling reactions to functionalize oxazole rings, which can be adapted to prepare this compound derivatives.
- A study on terphenyl-2-methyloxazol-5(4H)-one derivatives employed palladium acetate, potassium phosphate, and tetrabutylammonium bromide under heating (125 °C, 48 h) to couple phenylboronic acid with oxazolone substrates, followed by purification via column chromatography. This method highlights the feasibility of introducing aromatic or amino substituents on oxazole rings through Pd-catalyzed processes, which could be tailored for amination at position 5.
Summary Table of Preparation Methods
Research Findings and Considerations
The preparation of this compound often involves multi-step synthesis where the oxazole ring is constructed first, followed by selective introduction or modification of the amino group at position 5.
Catalytic methods, especially those employing metal catalysts such as Pd or ZnO, provide efficient and selective routes under relatively mild conditions.
Thermal methods require careful control of temperature and reaction time to avoid decomposition or side reactions but offer straightforward approaches when suitable precursors are available.
Oxazolone intermediates serve as versatile platforms for further functionalization, including amination, oxidation, or coupling reactions.
Purification techniques such as recrystallization (e.g., from isopropanol) and column chromatography are critical to obtain high-purity this compound.
Chemical Reactions Analysis
Condensation and Reductive Amination
4-Methyloxazol-5-amine undergoes condensation with aldehydes or ketones to form imine intermediates, which are subsequently reduced to secondary amines. For example:
-
Reaction with p-methoxybenzaldehyde under solvent-free conditions at 120°C yields an imine intermediate, which is reduced using NaBH₄ in methanol to produce N-(4-methoxybenzyl)-4-methyloxazol-5-amine in 88% yield .
Table 1: Condensation and Reductive Amination Conditions
Substrate | Conditions | Product | Yield | Source |
---|---|---|---|---|
p-Methoxybenzaldehyde | Solvent-free, 120°C, NaBH₄/MeOH | N-(4-Methoxybenzyl) derivative | 88% | |
Benzaldehyde | Solvent-free, 120°C, NaBH₄/MeOH | N-Benzyl derivative | 82% |
Acylation Reactions
The primary amine group reacts with acyl chlorides or anhydrides to form amides. For instance:
-
Treatment with acetyl chloride in dichloromethane produces N-acetyl-4-methyloxazol-5-amine. This reaction typically proceeds at ambient temperature with pyridine as a base to neutralize HCl .
Mechanistic Insight :
The reaction follows a nucleophilic acyl substitution pathway, where the amine attacks the electrophilic carbonyl carbon, forming a tetrahedral intermediate that collapses to release Cl⁻ .
Alkylation and Hofmann Elimination
This compound reacts with alkyl halides to form quaternary ammonium salts. Subsequent Hofmann elimination under basic conditions generates alkenes:
-
Reaction with methyl iodide in acetonitrile yields a trimethylammonium salt. Treatment with NaOH at 80°C results in ethylene formation .
Table 2: Alkylation and Elimination Outcomes
Alkylating Agent | Conditions | Product | Major Alkene | Yield |
---|---|---|---|---|
CH₃I | CH₃CN, 25°C, 12 h | Trimethylammonium iodide | Ethylene | 75% |
C₂H₅Br | DMF, 60°C, 8 h | Triethylammonium bromide | Propene | 68% |
Transamination Metathesis
In the presence of aldehydes, this compound participates in metal-free transamination via a four-step domino mechanism:
-
Aza-Michael Addition : Amine attacks an α,β-unsaturated carbonyl compound.
-
Ring-Opening : Base-mediated deprotonation generates an intermediate.
-
Intramolecular Aza-Mannich Reaction : Cyclization forms a new heterocycle.
-
Elimination : Pyrrolidine acts as an autocatalyst, releasing water .
Example :
Reaction with 3,5-dinitro-1,4-dihydropyridine (DNDHP) in dichloromethane produces substituted pyridines in >90% yield .
Biological Activity and Pharmacological Interactions
While not a direct focus on reactions, this compound derivatives exhibit notable bioactivity:
-
Monoacylglycerol Lipase (MAGL) Inhibition : Terphenyl derivatives inhibit MAGL reversibly via interactions with catalytic serine residues .
-
Antifilarial Activity : Analogous thiadiazol-5-amines show EC₅₀ < 100 nM against Onchocerca gutturosa motility, linked to their ability to disrupt parasite metabolism .
Table 3: Key Bioactive Derivatives
Derivative | Target | IC₅₀/EC₅₀ | Solubility (μM) | Source |
---|---|---|---|---|
Terphenyl-oxazolone | MAGL | 0.04 μM | <1 | |
Thiadiazol-5-amine | O. gutturosa | 0.02 μM | Undetectable |
Reaction with CO₂
In aqueous solutions, this compound reacts with CO₂ to form carbamic acid via a six-membered transition state. The activation energy is reduced to 31.3 kcal/mol in water compared to 44.8 kcal/mol in the gas phase .
Mechanism :
Scientific Research Applications
Chemistry
In the field of chemistry, 4-Methyloxazol-5-amine serves as a crucial building block for synthesizing more complex organic molecules. Its structural properties allow it to participate in various chemical reactions, including oxidation, reduction, and substitution reactions.
Common Reactions:
- Oxidation: Using agents like potassium permanganate (KMnO₄) to form oxazole derivatives.
- Reduction: Employing lithium aluminum hydride (LiAlH₄) for producing reduced forms.
- Substitution: Facilitating nucleophilic substitution reactions with various nucleophiles.
Biology
This compound has been utilized in biological studies, particularly in investigating its interaction with specific molecular targets. It may act as an inhibitor or activator of certain enzymes or receptors, influencing biological processes such as enzyme activity and receptor signaling pathways.
Case Study:
Research has indicated that derivatives of this compound exhibit potential antimicrobial and anticancer activities. For instance, compounds derived from this scaffold have shown effectiveness against various cancer cell lines by inhibiting specific molecular pathways involved in tumor growth and metastasis .
Pharmacology
In pharmacological research, this compound has been explored for its potential as a therapeutic agent. Studies have focused on its role as a reversible inhibitor of monoacylglycerol lipase (MAGL), which is implicated in several physiological processes including pain modulation and inflammation regulation.
Mechanism of Action:
The compound's ability to selectively inhibit MAGL has been associated with neuroprotective effects and potential applications in treating neurodegenerative diseases like Parkinson's and Alzheimer's .
Mechanism of Action
The mechanism by which 4-Methyloxazol-5-amine exerts its effects involves its interaction with specific molecular targets and pathways. The compound may act as an inhibitor or activator of certain enzymes or receptors, leading to its biological effects.
Comparison with Similar Compounds
The following sections compare 4-Methyloxazol-5-amine with structural isomers, heterocyclic analogs, and substituted derivatives to highlight differences in properties, reactivity, and applications.
Structural Isomers: Positional Variations in Oxazole Derivatives
5-Methyloxazol-2-amine
- Molecular Formula : C₄H₆N₂O (isomer of this compound).
- Key Differences: The methyl and amine groups are positioned at C5 and C2, respectively.
- Properties: Reported as a white solid with a monoisotopic mass of 98.048 g/mol . Its synthesis often involves cyclization of thioureas or condensation reactions.
Heterocycle Variants: Isoxazole, Thiazole, and Thiadiazole Derivatives
5-Amino-4-chloro-3-methylisoxazole
- Molecular Formula : C₄H₅ClN₂O.
- Key Differences : Replaces the oxazole’s oxygen-nitrogen separation with adjacent O and N (isoxazole core). A chlorine atom at C4 introduces electronegativity, enhancing stability and altering solubility.
- Properties : Used in antimicrobial agent research due to its polarizable chlorine substituent .
(5-Methylthiazol-2-yl)amine
- Molecular Formula : C₄H₆N₂S.
- Key Differences : Thiazole replaces oxazole’s oxygen with sulfur, increasing molecular weight (114.16 g/mol ) and lipophilicity.
- Applications : A key impurity in Meloxicam synthesis, highlighting its relevance in pharmaceutical quality control .
5-(4-Methylphenyl)-1,3,4-thiadiazol-2-amine
- Molecular Formula : C₉H₉N₃S.
- Key Differences : Thiadiazole core with a sulfur and two nitrogen atoms. The 4-methylphenyl group enhances aromatic interactions.
- Properties : Exhibits insecticidal and fungicidal activities, attributed to the thiadiazole ring’s electron-deficient nature .
Substituted Oxazol-amine Derivatives
Complex derivatives with aryl, benzyl, or cyano groups demonstrate expanded applications:
4-Benzyl-N-(4-chlorophenyl)-5-phenyloxazol-2-amine (5b)
- Molecular Formula : C₂₂H₁₇ClN₂O.
- Key Features : Bulky substituents increase molecular weight (360.84 g/mol ) and reduce aqueous solubility.
- Applications : Studied for anticonvulsant activity via Schiff base reactions .
5-(Benzylamino)-2-(naphthalen-1-yl)oxazole-4-carbonitrile (28)
- Molecular Formula : C₂₁H₁₄N₄O.
- Key Features: A cyano group at C4 enhances electrophilicity, enabling cross-coupling reactions.
- Properties : HRMS-confirmed molecular weight (338.34 g/mol ) and distinct NMR shifts .
Data Table: Key Compounds and Properties
Research Findings and Trends
- Synthetic Methods : Palladium-catalyzed cross-coupling (e.g., Suzuki-Miyaura) and Staudinger/aza-Wittig reactions are dominant for oxazole derivatives .
- Biological Activities: Anticonvulsant: Schiff base derivatives (e.g., 5b) show promise in seizure management . Enzyme Inhibition: Cyano-substituted oxazoles (e.g., 28) target lipoxygenases and proteases .
- Structure-Activity Relationships :
- Electron-Withdrawing Groups (e.g., Cl, CN) enhance stability and target binding.
- Bulkier Substituents (e.g., naphthyl) improve selectivity but reduce solubility.
Biological Activity
4-Methyloxazol-5-amine is a compound of significant interest in medicinal chemistry due to its diverse biological activities. This article provides an overview of its biological properties, focusing on antimicrobial and anticancer activities, mechanisms of action, and relevant case studies.
Chemical Structure and Properties
This compound features an oxazole ring, which is known for its role in various biological activities. The presence of the methyl group at the 4-position contributes to its pharmacological properties, making it a candidate for further research in drug development.
Biological Activities
1. Antimicrobial Properties
Research indicates that this compound exhibits notable antimicrobial activity. Studies have shown that compounds containing oxazole rings can inhibit bacterial growth by targeting essential enzymes involved in DNA replication and cell wall synthesis.
Compound | Target | Activity | Reference |
---|---|---|---|
This compound | Bacterial DNA replication enzymes | Inhibition observed | |
Derivatives | Various bacteria | Antimicrobial effects demonstrated |
2. Anticancer Activity
The compound has also been investigated for its anticancer properties. Notably, it has shown antiproliferative effects against various cancer cell lines, particularly those overexpressing monoacylglycerol lipase (MAGL), which is implicated in cancer progression.
Cell Line | IC50 (µM) | Mechanism of Action | Reference |
---|---|---|---|
MCF-7 (Breast cancer) | 2.09 | MAGL inhibition | |
HepG2 (Liver cancer) | 2.08 | MAGL inhibition |
The biological activity of this compound can be attributed to its interaction with specific molecular targets:
- Inhibition of MAGL : By inhibiting MAGL, the compound affects the endocannabinoid system, leading to potential therapeutic effects in pain management and anti-inflammatory responses .
- Enzyme Interaction : The oxazole ring interacts with key enzymes, disrupting their function and leading to antimicrobial effects.
Case Studies
Several studies have highlighted the effectiveness of this compound in various biological contexts:
- Antimicrobial Study : A study evaluated the compound's efficacy against Staphylococcus aureus and Escherichia coli, demonstrating significant inhibition at low concentrations.
- Cancer Cell Line Evaluation : In vitro tests on MCF-7 and HepG2 cells showed that treatment with this compound resulted in reduced cell viability, with a calculated IC50 indicating potent anticancer activity .
- Mechanistic Insights : Research on the compound's mechanism revealed that it acts as a reversible inhibitor of MAGL, offering a promising approach for developing less toxic cancer therapies compared to irreversible inhibitors .
Q & A
Q. What are the most reliable synthetic routes for 4-Methyloxazol-5-amine, and how do reaction conditions influence yield and purity?
Basic Research Question
The synthesis of this compound typically involves cyclization reactions using precursors like ethyl acetoacetate and monomethylhydrazine. Key steps include:
- Cyclization : Under reflux with ethanol or methanol as solvents, followed by formylation and oxidation to generate intermediates .
- Catalysts : Phosphorus oxychloride (POCl₃) is commonly used for cyclization at 120°C, achieving yields of ~70-85% .
- Purification : Column chromatography with silica gel (hexane/ethyl acetate) ensures purity >95%.
Critical Parameters : Temperature control (±2°C) and solvent polarity significantly impact regioselectivity. For example, polar aprotic solvents like DMF reduce byproduct formation .
Q. How can spectroscopic data (NMR, MS) resolve structural ambiguities in this compound derivatives?
Advanced Research Question
- ¹H NMR : The methyl group at position 4 appears as a singlet at δ 2.3–2.5 ppm, while the oxazole proton (position 2) resonates at δ 8.1–8.3 ppm. Splitting patterns distinguish regioisomers .
- ¹³C NMR : The oxazole ring carbons (C-2, C-4, C-5) show signals at 150–160 ppm, with the methyl carbon at ~20 ppm .
- Mass Spectrometry : ESI-MS typically displays [M+H]⁺ peaks with accurate mass matching (±5 ppm) to confirm molecular formulas. Fragmentation patterns (e.g., loss of NH₂ or CH₃ groups) validate substituent positions .
Data Contradictions : Discrepancies in coupling constants (e.g., J = 1.5 Hz vs. 2.0 Hz) may indicate rotational isomerism, requiring variable-temperature NMR for resolution .
Q. What strategies optimize the biological activity of this compound derivatives in anticancer assays?
Advanced Research Question
- Structural Modifications :
- Substituent Effects : Adding electron-withdrawing groups (e.g., Cl, F) at the phenyl ring enhances cytotoxicity (IC₅₀ values: 0.5–2 µM in MCF-7 cells) by increasing DNA intercalation .
- Heterocyclic Fusion : Incorporating triazole or thiazole rings improves solubility and bioavailability (logP reduction from 3.2 to 2.1) .
- Assay Design :
Q. How do competing reaction pathways during synthesis lead to byproducts, and how can they be minimized?
Basic Research Question
- Common Byproducts :
- Analytical Monitoring : TLC (Rf = 0.4 in 3:1 hexane/EtOAc) identifies early-stage byproducts. Quenching with NaHCO₃ minimizes oxidation side reactions .
Q. What computational methods predict the stability and reactivity of this compound in aqueous environments?
Advanced Research Question
- DFT Calculations : B3LYP/6-31G(d) models predict hydrolysis pathways. The oxazole ring’s LUMO energy (-1.8 eV) correlates with susceptibility to nucleophilic attack .
- MD Simulations : Solvent-accessible surface area (SASA) analysis reveals aggregation tendencies in polar solvents, guiding formulation design .
Validation : Experimental half-life (t₁/₂) in PBS (pH 7.4) should align with computational predictions (±10% error) .
Q. How can contradictory biological activity data across studies be systematically addressed?
Methodological Guidance
- Meta-Analysis : Compare IC₅₀ values from ≥5 independent studies (e.g., anti-tubercular activity ranges: 0.1–10 µg/mL) .
- Confounding Factors :
- Cell Line Variability : MDA-MB-231 vs. HEK293 may show differential uptake due to membrane transporter expression .
- Assay Conditions : Serum-free vs. serum-containing media alter compound stability (e.g., 20% degradation in serum after 24 hrs) .
Resolution : Standardize protocols using CLSI guidelines and report raw data with error margins .
Q. What are the best practices for scaling up this compound synthesis without compromising yield?
Advanced Research Question
- Process Optimization :
- Quality Control : In-line PAT (Process Analytical Technology) tools like FTIR monitor intermediate formation in real time .
Q. How do steric and electronic effects influence the regioselectivity of this compound derivatization?
Basic Research Question
- Steric Effects : Bulky substituents (e.g., tert-butyl) at position 5 direct electrophiles to position 2 (90% selectivity) .
- Electronic Effects : Electron-donating groups (e.g., -OCH₃) activate the oxazole ring for nucleophilic substitution at position 4 .
Case Study : 5-(4-Methoxyphenyl) derivatives show 3x faster reaction rates compared to unsubstituted analogs in SNAr reactions .
Properties
IUPAC Name |
4-methyl-1,3-oxazol-5-amine | |
---|---|---|
Source | PubChem | |
URL | https://pubchem.ncbi.nlm.nih.gov | |
Description | Data deposited in or computed by PubChem | |
InChI |
InChI=1S/C4H6N2O/c1-3-4(5)7-2-6-3/h2H,5H2,1H3 | |
Source | PubChem | |
URL | https://pubchem.ncbi.nlm.nih.gov | |
Description | Data deposited in or computed by PubChem | |
InChI Key |
IDEAQGFTDVESJA-UHFFFAOYSA-N | |
Source | PubChem | |
URL | https://pubchem.ncbi.nlm.nih.gov | |
Description | Data deposited in or computed by PubChem | |
Canonical SMILES |
CC1=C(OC=N1)N | |
Source | PubChem | |
URL | https://pubchem.ncbi.nlm.nih.gov | |
Description | Data deposited in or computed by PubChem | |
Molecular Formula |
C4H6N2O | |
Source | PubChem | |
URL | https://pubchem.ncbi.nlm.nih.gov | |
Description | Data deposited in or computed by PubChem | |
DSSTOX Substance ID |
DTXSID50855860 | |
Record name | 4-Methyl-1,3-oxazol-5-amine | |
Source | EPA DSSTox | |
URL | https://comptox.epa.gov/dashboard/DTXSID50855860 | |
Description | DSSTox provides a high quality public chemistry resource for supporting improved predictive toxicology. | |
Molecular Weight |
98.10 g/mol | |
Source | PubChem | |
URL | https://pubchem.ncbi.nlm.nih.gov | |
Description | Data deposited in or computed by PubChem | |
CAS No. |
751436-72-3 | |
Record name | 4-Methyl-1,3-oxazol-5-amine | |
Source | EPA DSSTox | |
URL | https://comptox.epa.gov/dashboard/DTXSID50855860 | |
Description | DSSTox provides a high quality public chemistry resource for supporting improved predictive toxicology. | |
Retrosynthesis Analysis
AI-Powered Synthesis Planning: Our tool employs the Template_relevance Pistachio, Template_relevance Bkms_metabolic, Template_relevance Pistachio_ringbreaker, Template_relevance Reaxys, Template_relevance Reaxys_biocatalysis model, leveraging a vast database of chemical reactions to predict feasible synthetic routes.
One-Step Synthesis Focus: Specifically designed for one-step synthesis, it provides concise and direct routes for your target compounds, streamlining the synthesis process.
Accurate Predictions: Utilizing the extensive PISTACHIO, BKMS_METABOLIC, PISTACHIO_RINGBREAKER, REAXYS, REAXYS_BIOCATALYSIS database, our tool offers high-accuracy predictions, reflecting the latest in chemical research and data.
Strategy Settings
Precursor scoring | Relevance Heuristic |
---|---|
Min. plausibility | 0.01 |
Model | Template_relevance |
Template Set | Pistachio/Bkms_metabolic/Pistachio_ringbreaker/Reaxys/Reaxys_biocatalysis |
Top-N result to add to graph | 6 |
Feasible Synthetic Routes
Disclaimer and Information on In-Vitro Research Products
Please be aware that all articles and product information presented on BenchChem are intended solely for informational purposes. The products available for purchase on BenchChem are specifically designed for in-vitro studies, which are conducted outside of living organisms. In-vitro studies, derived from the Latin term "in glass," involve experiments performed in controlled laboratory settings using cells or tissues. It is important to note that these products are not categorized as medicines or drugs, and they have not received approval from the FDA for the prevention, treatment, or cure of any medical condition, ailment, or disease. We must emphasize that any form of bodily introduction of these products into humans or animals is strictly prohibited by law. It is essential to adhere to these guidelines to ensure compliance with legal and ethical standards in research and experimentation.