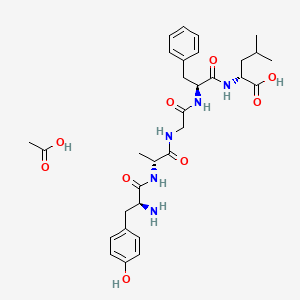
(d-Ala2,d-leu5)-enkephalin acetate
Description
(d-Ala2,d-leu5)-enkephalin acetate is a useful research compound. Its molecular formula is C31H43N5O9 and its molecular weight is 629.7 g/mol. The purity is usually 95%.
BenchChem offers high-quality this compound suitable for many research applications. Different packaging options are available to accommodate customers' requirements. Please inquire for more information about this compound including the price, delivery time, and more detailed information at info@benchchem.com.
Properties
IUPAC Name |
acetic acid;(2R)-2-[[(2S)-2-[[2-[[(2R)-2-[[(2S)-2-amino-3-(4-hydroxyphenyl)propanoyl]amino]propanoyl]amino]acetyl]amino]-3-phenylpropanoyl]amino]-4-methylpentanoic acid | |
---|---|---|
Source | PubChem | |
URL | https://pubchem.ncbi.nlm.nih.gov | |
Description | Data deposited in or computed by PubChem | |
InChI |
InChI=1S/C29H39N5O7.C2H4O2/c1-17(2)13-24(29(40)41)34-28(39)23(15-19-7-5-4-6-8-19)33-25(36)16-31-26(37)18(3)32-27(38)22(30)14-20-9-11-21(35)12-10-20;1-2(3)4/h4-12,17-18,22-24,35H,13-16,30H2,1-3H3,(H,31,37)(H,32,38)(H,33,36)(H,34,39)(H,40,41);1H3,(H,3,4)/t18-,22+,23+,24-;/m1./s1 | |
Source | PubChem | |
URL | https://pubchem.ncbi.nlm.nih.gov | |
Description | Data deposited in or computed by PubChem | |
InChI Key |
YRZBXSPTRALQEX-IIKZURRYSA-N | |
Source | PubChem | |
URL | https://pubchem.ncbi.nlm.nih.gov | |
Description | Data deposited in or computed by PubChem | |
Canonical SMILES |
CC(C)CC(C(=O)O)NC(=O)C(CC1=CC=CC=C1)NC(=O)CNC(=O)C(C)NC(=O)C(CC2=CC=C(C=C2)O)N.CC(=O)O | |
Source | PubChem | |
URL | https://pubchem.ncbi.nlm.nih.gov | |
Description | Data deposited in or computed by PubChem | |
Isomeric SMILES |
C[C@H](C(=O)NCC(=O)N[C@@H](CC1=CC=CC=C1)C(=O)N[C@H](CC(C)C)C(=O)O)NC(=O)[C@H](CC2=CC=C(C=C2)O)N.CC(=O)O | |
Source | PubChem | |
URL | https://pubchem.ncbi.nlm.nih.gov | |
Description | Data deposited in or computed by PubChem | |
Molecular Formula |
C31H43N5O9 | |
Source | PubChem | |
URL | https://pubchem.ncbi.nlm.nih.gov | |
Description | Data deposited in or computed by PubChem | |
DSSTOX Substance ID |
DTXSID70746721 | |
Record name | L-Tyrosyl-D-alanylglycyl-L-phenylalanyl-D-leucine--acetic acid (1/1) | |
Source | EPA DSSTox | |
URL | https://comptox.epa.gov/dashboard/DTXSID70746721 | |
Description | DSSTox provides a high quality public chemistry resource for supporting improved predictive toxicology. | |
Molecular Weight |
629.7 g/mol | |
Source | PubChem | |
URL | https://pubchem.ncbi.nlm.nih.gov | |
Description | Data deposited in or computed by PubChem | |
CAS No. |
94825-57-7 | |
Record name | L-Tyrosyl-D-alanylglycyl-L-phenylalanyl-D-leucine--acetic acid (1/1) | |
Source | EPA DSSTox | |
URL | https://comptox.epa.gov/dashboard/DTXSID70746721 | |
Description | DSSTox provides a high quality public chemistry resource for supporting improved predictive toxicology. | |
Retrosynthesis Analysis
AI-Powered Synthesis Planning: Our tool employs the Template_relevance Pistachio, Template_relevance Bkms_metabolic, Template_relevance Pistachio_ringbreaker, Template_relevance Reaxys, Template_relevance Reaxys_biocatalysis model, leveraging a vast database of chemical reactions to predict feasible synthetic routes.
One-Step Synthesis Focus: Specifically designed for one-step synthesis, it provides concise and direct routes for your target compounds, streamlining the synthesis process.
Accurate Predictions: Utilizing the extensive PISTACHIO, BKMS_METABOLIC, PISTACHIO_RINGBREAKER, REAXYS, REAXYS_BIOCATALYSIS database, our tool offers high-accuracy predictions, reflecting the latest in chemical research and data.
Strategy Settings
Precursor scoring | Relevance Heuristic |
---|---|
Min. plausibility | 0.01 |
Model | Template_relevance |
Template Set | Pistachio/Bkms_metabolic/Pistachio_ringbreaker/Reaxys/Reaxys_biocatalysis |
Top-N result to add to graph | 6 |
Feasible Synthetic Routes
Q1: How does DADLE interact with its target and what are the downstream effects observed in cardiomyocytes?
A1: DADLE specifically binds to δ-opioid receptors in cardiomyocytes [, ]. This binding triggers a cascade of intracellular signaling events, notably involving the activation of extracellular signal-regulated kinase (ERK) and phosphatidylinositol 3-kinase (PI3K) pathways [, ]. These pathways play a crucial role in promoting cell survival by increasing the levels of survivin and Bcl-2, proteins known for their anti-apoptotic properties [, ]. Furthermore, DADLE's activation of δ-opioid receptors leads to a decrease in Bax translocation, a process associated with apoptosis initiation [, ]. This protective effect against apoptosis also involves reducing mitochondrial membrane damage and decreasing the production of reactive oxygen species (ROS) [, ].
Q2: Can DADLE exert cardioprotective effects in diabetic hearts, and if not, what mechanisms are involved?
A2: Research indicates that DADLE's cardioprotective effects are significantly diminished in diabetic hearts [, ]. This resistance is attributed to the impairment of the phosphatidylinositol 3-kinase (PI3K)-Akt signaling pathway, a pathway crucial for mediating cardioprotection [, ]. The underlying cause of this impairment appears to be linked to an angiotensin II type 1 (AT1) receptor-mediated upregulation of calcineurin activity in diabetic hearts [, ]. This heightened calcineurin activity disrupts the phosphorylation of Jak2, a key upstream component of the PI3K-Akt pathway, ultimately hindering DADLE's ability to confer cardioprotection in the diabetic setting [, ].
Q3: Beyond cardiomyocytes, are there other areas where DADLE's interaction with opioid receptors has been investigated?
A3: Yes, research has explored DADLE's effects on opioid receptors in other systems, including the modulation of muscle contractions in the rat ileum []. While the provided abstract doesn't delve into specific results, it suggests that understanding DADLE's influence on different opioid receptor subtypes in the ileum could be relevant for gastrointestinal pharmacology. This highlights that DADLE's actions extend beyond the cardiovascular system, prompting further research into its potential therapeutic applications in areas like gastrointestinal disorders.
Disclaimer and Information on In-Vitro Research Products
Please be aware that all articles and product information presented on BenchChem are intended solely for informational purposes. The products available for purchase on BenchChem are specifically designed for in-vitro studies, which are conducted outside of living organisms. In-vitro studies, derived from the Latin term "in glass," involve experiments performed in controlled laboratory settings using cells or tissues. It is important to note that these products are not categorized as medicines or drugs, and they have not received approval from the FDA for the prevention, treatment, or cure of any medical condition, ailment, or disease. We must emphasize that any form of bodily introduction of these products into humans or animals is strictly prohibited by law. It is essential to adhere to these guidelines to ensure compliance with legal and ethical standards in research and experimentation.