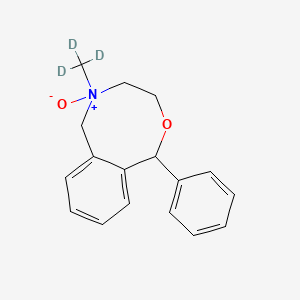
Nefopam-d3 N-Oxide
- Click on QUICK INQUIRY to receive a quote from our team of experts.
- With the quality product at a COMPETITIVE price, you can focus more on your research.
Overview
Description
Nefopam-d3 N-Oxide is a deuterated metabolite of the non-opioid analgesic nefopam. This compound (CAS 1346603-50-6) is isotopically labeled with three deuterium atoms at the methyl group, resulting in a molecular formula of C₁₇H₁₆D₃NO₂ and a molecular weight of 272.36 g/mol . This deuterated form is primarily utilized as an internal standard in pharmacokinetic (PK) studies to enhance analytical precision via mass spectrometry .
Nefopam undergoes hepatic metabolism to produce two major metabolites: N-desmethylnefopam and Nefopam N-Oxide (CAS 66091-32-5; MW 269.35 g/mol) . The N-Oxide derivative is formed via oxidation of the tertiary amine group, a common metabolic pathway for xenobiotics. The deuterated variant, this compound, shares structural and functional similarities with its non-deuterated counterpart but offers improved stability in tracer studies .
Preparation Methods
Synthetic Routes and Reaction Conditions
The synthesis of Nefopam-d3 N-Oxide involves the incorporation of deuterium into the Nefopam N-Oxide molecule. This is typically achieved through a series of chemical reactions that replace hydrogen atoms with deuterium. The exact synthetic route and reaction conditions can vary, but generally involve the use of deuterated reagents and solvents under controlled conditions .
Industrial Production Methods
Industrial production of this compound follows similar principles as laboratory synthesis but on a larger scale. This involves the use of specialized equipment to handle deuterated compounds and ensure the purity and consistency of the final product. The production process is designed to be efficient and cost-effective while maintaining high standards of quality control .
Chemical Reactions Analysis
Oxidation Mechanism
The tertiary amine in Nefopam undergoes oxidation to form the N-oxide via electrophilic attack by KPMS. The reaction is chemoselective, favoring oxidation of more basic amines .
Fragmentation in Mass Spectrometry
The fragmentation patterns observed in LC-Q-ToF-MS highlight structural differences between Nefopam and its N-Oxide:
-
Nefopam : Major product ions at m/z 181 (loss of C₃H₇NO) and m/z 179 (further loss of 2H) .
-
Nefopam N-Oxide : Additional cleavage of C₃H₉NO from the protonated molecular ion (m/z 270), yielding m/z 195 .
Mass Spectrometry
The exact mass of Nefopam-d3 N-Oxide ([M + H]⁺) was measured as 270.1491 , with a mass accuracy of 1.11 ppm , confirming its molecular formula (C₁₇H₁₉NO₂) .
FT-IR Spectroscopy
Characteristic N-O group vibrations in the FT-IR spectra (bands near 928 cm⁻¹ ) validate the N-oxide functionality .
Comparison with Parent Compound
Property | Nefopam | This compound |
---|---|---|
Molecular Formula | C₁₇H₁₉NO | C₁₇H₁₆NO₂D₃ |
Molecular Weight | 254.15 g/mol | 272.36 g/mol |
Key Functional Group | Tertiary amine | N-Oxide |
Synthesis Reagents | KPMS | Deuterated reagents + KPMS |
Mechanistic Insights
The N-O bond in this compound introduces polarity and reactivity, enabling participation in redox processes. The bond order (slightly above 1) and backdonation effects (dependent on substituents) influence its stability and reactivity .
This compound’s well-defined synthesis, analytical characterization, and role in pharmacological research underscore its utility in advancing pain management therapies.
Scientific Research Applications
Nefopam-d3 N-Oxide is a deuterium-labeled derivative of Nefopam N-Oxide, which is known for its analgesic properties. Nefopam is a non-opioid, non-steroidal central analgesic that works by inhibiting the reuptake of serotonin and norepinephrine and by modulating voltage-sensitive calcium and sodium channels. The deuterium labeling of this compound makes it useful in pharmacological research, enabling more precise tracking in biological systems because deuterium has different physical properties than hydrogen.
Scientific Research Applications
This compound is used mainly in scientific research because of its unique properties.
Pharmacokinetic Studies
- The deuterium label allows the tracing and quantification of metabolic pathways.
- It is valuable in drug development and analytical chemistry compared to non-deuterated counterparts.
Biological Activity Studies
- This compound's biological activity is similar to that of Nefopam. Its mechanisms include:
- Inhibition of serotonin and norepinephrine reuptake
- Modulation of voltage-sensitive calcium and sodium channels
These actions lead to analgesic effects without the respiratory depression that is common with opioid analgesics, making it a candidate for pain management studies.
Interactions with Biological Systems
- This compound is used in studies that focus on its interactions with various biological systems. These studies may include:
- Receptor binding assays
- Enzyme inhibition studies
- Cellular signaling pathways
Such studies improve the understanding of how the compound can be used effectively in clinical settings.
Nefopam as an Analgesic
Nefopam is a clinically effective analgesic agent that is used to control mild to moderate pain, but its mechanism of action is not fully known . Studies have investigated the antinociceptive activity of nefopam using assays such as the mouse abdominal constriction assay and the tail immersion test .
- Nefopam was found to be potent against acetic acid-induced visceral nociception .
- Nefopam's dose-response relationships were shifted to the right in the presence of opioid receptor antagonists like naloxone or naltrindole .
- An inhibitor of neutral endopeptidase was able to potentiate nefopam’s antinociceptive activity .
- An alpha2-adrenoceptor antagonist shifted the dose-response curves for both clonidine and nefopam .
These data suggest that nefopam's antinociceptive activity involves opioidergic and noradrenergic components in the mouse abdominal constriction assay and tail immersion test .
Effects on Emergence Agitation
Nefopam infusion can prevent and reduce the severity of emergence agitation (EA) after nasal surgery, without delaying extubation .
- A study allocated patients randomly to either a nefopam group (20 mg of nefopam) or a control group (saline) .
- The overall incidence of EA was lower in the nefopam group compared to the control group (34% vs 54%) .
- The incidence of severe EA was also lower in the nefopam group (8% vs 38%) .
- Heart rate was higher in the nefopam group than in the control group from the end of surgery to 3 minutes after extubation .
- There were no significant differences in adverse events between the two groups .
Additional Studies and Findings
- Nefopam can inhibit calcium influx, cGMP formation, and NMDA-mediated excitotoxicity .
- Nefopam prevented N-methyl-D-aspartate (NMDA)-mediated excitotoxicity following stimulation of L-type voltage sensitive calcium channels .
- Neuroprotection was associated with a reduction in the BayK8644-induced increase in intracellular calcium concentration .
- Nefopam also inhibited intracellular cGMP formation .
- Intravenous nefopam infusion resulted in a significant reduction in postoperative pain scores and opioid requirements, while also decreasing opioid-related adverse effects .
- A meta-analysis indicated that intravenous nefopam infusion could significantly decrease opioid requirements .
- The use of nefopam could significantly reduce the incidence of nausea, vomiting, and pruritus .
- Nefopam hydrochloride has significant analgesic effects and can supplement morphine in postoperative pain management .
Mechanism of Action
Nefopam-d3 N-Oxide exerts its effects through mechanisms similar to those of Nefopam. It acts in the brain and spinal cord to relieve pain via:
Triple Monoamine Reuptake Inhibition: Inhibits the reuptake of serotonin, norepinephrine, and dopamine, enhancing their levels in the synaptic cleft.
Modulation of Glutamatergic Transmission: Reduces hyperalgesia by modulating glutamate receptors.
These actions result in potent analgesic effects without the respiratory depression commonly associated with opioid analgesics .
Comparison with Similar Compounds
Comparison with Structurally Similar Compounds
Nefopam and Its Metabolites
Parameter | Nefopam HCl | Nefopam N-Oxide | Nefopam-d3 N-Oxide | N-Desmethylnefopam HCl |
---|---|---|---|---|
CAS Number | 23327-57-3 | 66091-32-5 | 1346603-50-6 | 147656-98-2 |
Molecular Formula | C₁₇H₁₉NO | C₁₇H₁₉NO₂ | C₁₇H₁₆D₃NO₂ | C₁₆H₁₇NO |
Molecular Weight | 253.35 g/mol | 269.35 g/mol | 272.36 g/mol | 239.32 g/mol |
Key Functional Group | Tertiary amine | N-Oxide | Deuterated N-Oxide | Secondary amine |
Role in PK Studies | Parent drug | Major metabolite | Internal standard | Major metabolite |
Key Differences :
- This compound vs. Nefopam N-Oxide : The deuterated form has a 3 Da higher molecular weight due to isotopic substitution, which minimizes interference in mass spectrometry .
- N-Oxide vs. N-Desmethylnefopam : The N-Oxide retains the methyl group but introduces an oxygen atom, whereas N-desmethylnefopam loses the methyl group entirely. This structural divergence leads to differences in polarity, solubility, and metabolic clearance .
Comparison with Other N-Oxide Compounds
N-Oxide derivatives are common in drug metabolism. Examples include Sorafenib N-Oxide and plant alkaloids like Antofin N-Oxide (from Ficus septica) .
Pharmacokinetic Profiles: Nefopam N-Oxide vs. Sorafenib N-Oxide
Key Insights :
- Sorafenib N-Oxide exhibits significant brain penetration, with a 51.4% higher Cmax and 50.9% higher AUC in brain tissue compared to plasma .
Pharmacokinetic and Analytical Considerations
Metabolic Pathways
Nefopam is metabolized via N-oxidation and N-demethylation, with N-Oxide formation being a minor pathway compared to N-desmethylnefopam . A mixed-effects PK model for nefopam and its metabolites revealed:
- Simultaneous compartmental modeling : Nefopam follows two-compartment kinetics, while metabolites fit single-compartment models .
- Presystemic metabolism : Likely contributes to variable bioavailability .
Role of Deuterated Analogs
This compound is critical for quantifying the non-deuterated metabolite in biological matrices. Its use minimizes ion suppression and matrix effects in LC-MS/MS assays, improving accuracy .
Biological Activity
Nefopam-d3 N-Oxide is a deuterium-labeled derivative of Nefopam, a non-opioid analgesic known for its efficacy in pain relief. This compound exhibits biological activities similar to its parent compound, with additional advantages due to the incorporation of deuterium, which enhances tracking and quantification in pharmacological studies. This article explores the biological activity of this compound, focusing on its mechanisms of action, pharmacokinetics, and relevant case studies.
This compound operates through several mechanisms that contribute to its analgesic properties:
- Triple Monoamine Reuptake Inhibition : It inhibits the reuptake of serotonin, norepinephrine, and dopamine, which plays a critical role in modulating pain perception.
- Modulation of Glutamatergic Transmission : The compound affects glutamate release and receptor activity, potentially mitigating excitotoxicity associated with various neurological disorders .
- Calcium and Sodium Channel Modulation : It influences voltage-sensitive calcium and sodium channels, further contributing to its analgesic effects without inducing respiratory depression typical of opioid analgesics.
Pharmacokinetics
The deuterium labeling in this compound allows for enhanced pharmacokinetic studies. The distinct physical properties of deuterium facilitate precise tracking in biological systems, making it valuable for understanding absorption, distribution, metabolism, and excretion (ADME) profiles.
Table 1: Pharmacokinetic Properties of this compound
Property | Description |
---|---|
Molecular Formula | C17H16D3NO2 |
Molecular Weight | 272.36 g/mol |
Bioavailability | High (specific values vary by study) |
Half-life | Varies based on dosage and method |
Study on Neurological Effects
A significant study investigated the effects of Nefopam on locomotor activity and neuroprotection in Wistar rats. The study found that administration of Nefopam at various doses (1.0 to 40 mg/kg) significantly influenced dopamine levels in the striatum and reduced L-DOPA content in critical brain regions. Notably:
- Locomotor Activity : Doses of 10 mg/kg reduced stereotypy and improved motor coordination compared to control groups.
- Neuroprotective Effects : The compound demonstrated potential in protecting dopaminergic neurons from excitotoxic damage associated with conditions like Parkinson’s disease .
Table 2: Effects of Nefopam on Neurotransmitter Levels
Dose (mg/kg) | DA Release (%) | L-DOPA Content Change (%) |
---|---|---|
1.0 | +50 | -10 |
10 | +206 | -25 |
20 | +150 | -30 |
40 | +100 | -40 |
Research Applications
This compound is primarily utilized in research settings due to its unique properties. Applications include:
- Pharmacological Studies : Understanding the interactions between Nefopam and various neurotransmitter systems.
- Clinical Research : Investigating its potential as an analgesic alternative in pain management protocols.
- Analytical Chemistry : Using its deuterium label for tracing metabolic pathways and drug interactions .
Q & A
Basic Research Questions
Q. What validated analytical methods are recommended for quantifying Nefopam-d3 N-Oxide in complex biological matrices?
- Methodological Answer : Use ultra-high-performance liquid chromatography tandem mass spectrometry (UHPLC-MS/MS) coupled with online solid-phase extraction (SPE) for high sensitivity and reduced matrix interference. Calibration curves should employ deuterated internal standards to correct for ion suppression/enhancement. For example, linear weighted regression (r² > 0.99) and signal-to-noise ratios >10 at lower limits of quantification (LLOQ) ensure precision . Include validation parameters such as accuracy (85–115%), intraday/interday variability (<15%), and recovery rates in spiked matrices.
Q. How should experimental designs be structured to assess the pharmacological activity of this compound?
- Methodological Answer : Employ dose-response studies with negative controls (e.g., vehicle-only groups) and positive controls (e.g., known NMDA receptor antagonists like MK-801). Use two-way ANOVA to isolate treatment effects from confounding variables (e.g., time-dependent metabolic changes). Ensure blinding during data collection to minimize bias. Reference compounds like orphenadrine can serve as structural analogs for comparative efficacy analysis .
Q. What statistical approaches are optimal for analyzing dose-response data in preclinical studies of this compound?
- Methodological Answer : Apply one-way or two-way ANOVA followed by post-hoc tests (e.g., Fisher’s LSD) to compare individual dose groups. Normalize data using logarithmic transformations if variances are unequal (Levene’s test) or distributions are non-normal (Shapiro-Wilk test). Report effect sizes and confidence intervals to contextualize significance thresholds (e.g., p ≤ 0.05) .
Advanced Research Questions
Q. How can computational models evaluate structural alerts for mutagenicity in this compound?
- Methodological Answer : Use (quantitative) structure-activity relationship [(Q)SAR] models to screen aromatic N-oxide substructures. Prioritize substructures linked to DNA-reactive mutagenicity (e.g., benzo[c][1,2,5]oxadiazole 1-oxide subclass). Cross-validate predictions with Ames test data and proprietary mutagenicity databases. Downgrade general alerts if public data show >20% non-mutagenic compounds in the subclass .
Q. What methodologies resolve discrepancies in pharmacokinetic data for this compound across independent studies?
- Methodological Answer : Conduct meta-analyses using mixed-effects models to account for inter-study variability (e.g., differences in dosing regimens or species-specific metabolism). Stratify data by covariates like administration route (oral vs. intravenous) or matrix type (plasma vs. tissue). Apply sensitivity analyses to identify outliers driven by methodological artifacts (e.g., incomplete SPE cleanup) .
Q. How do environmental factors influence the stability and metabolite formation of this compound in ecological studies?
- Methodological Answer : Design microcosm experiments simulating soil pH, temperature, and microbial activity gradients. Quantify N-oxide degradation products via stable isotope tracing (e.g., ¹⁵N-labeled analogs). Use linear mixed-effect (LME) models to assess interactions between environmental variables and degradation rates. Include negative controls (sterilized matrices) to distinguish biotic vs. abiotic pathways .
Q. What strategies ensure reproducibility when synthesizing and characterizing this compound?
- Methodological Answer : Document synthetic routes with detailed reaction conditions (e.g., solvent purity, catalyst ratios, and inert atmosphere protocols). Characterize intermediates via nuclear magnetic resonance (NMR) and high-resolution mass spectrometry (HRMS). Share raw spectral data in supplementary materials and adhere to FAIR (Findable, Accessible, Interoperable, Reusable) principles for data deposition .
Q. Guidance for Data Interpretation and Reporting
- Addressing Contradictory Findings : Use sensitivity analysis to test if conflicting results arise from methodological differences (e.g., extraction efficiency variations). For example, compare manual vs. automated SPE workflows in UHPLC-MS/MS assays .
- Systematic Literature Reviews : Follow PRISMA guidelines to screen databases (e.g., Embase, MEDLINE) using Boolean queries combining "this compound" with terms like "pharmacokinetics" or "mutagenicity." Map evidence gaps using tools like ROSES for ecological studies .
Properties
Molecular Formula |
C17H19NO2 |
---|---|
Molecular Weight |
272.36 g/mol |
IUPAC Name |
5-oxido-1-phenyl-5-(trideuteriomethyl)-1,3,4,6-tetrahydro-2,5-benzoxazocin-5-ium |
InChI |
InChI=1S/C17H19NO2/c1-18(19)11-12-20-17(14-7-3-2-4-8-14)16-10-6-5-9-15(16)13-18/h2-10,17H,11-13H2,1H3/i1D3 |
InChI Key |
ADOSMCNCNFMWSW-FIBGUPNXSA-N |
Isomeric SMILES |
[2H]C([2H])([2H])[N+]1(CCOC(C2=CC=CC=C2C1)C3=CC=CC=C3)[O-] |
Canonical SMILES |
C[N+]1(CCOC(C2=CC=CC=C2C1)C3=CC=CC=C3)[O-] |
Origin of Product |
United States |
Disclaimer and Information on In-Vitro Research Products
Please be aware that all articles and product information presented on BenchChem are intended solely for informational purposes. The products available for purchase on BenchChem are specifically designed for in-vitro studies, which are conducted outside of living organisms. In-vitro studies, derived from the Latin term "in glass," involve experiments performed in controlled laboratory settings using cells or tissues. It is important to note that these products are not categorized as medicines or drugs, and they have not received approval from the FDA for the prevention, treatment, or cure of any medical condition, ailment, or disease. We must emphasize that any form of bodily introduction of these products into humans or animals is strictly prohibited by law. It is essential to adhere to these guidelines to ensure compliance with legal and ethical standards in research and experimentation.