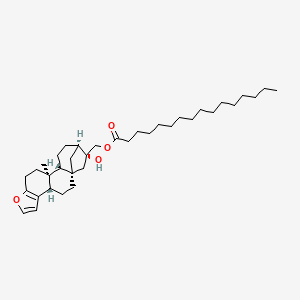
Cafestol palmitate
Overview
Description
Cafestol palmitate is a naturally occurring diterpene ester found in coffee beans, particularly in unfiltered coffee brews such as Turkish coffee, Scandinavian boiled coffee, and French-press coffee. It is known for its potential health benefits and bioactivities, including anti-inflammatory, anticancer, and cholesterol-lowering effects.
Preparation Methods
Synthetic Routes and Reaction Conditions: Cafestol palmitate can be synthesized through the esterification of cafestol with palmitic acid. The reaction typically involves heating cafestol with palmitic acid in the presence of a strong acid catalyst, such as sulfuric acid, under reflux conditions.
Industrial Production Methods: In industrial settings, this compound is often extracted from coffee beans using organic solvents like diethyl ether or tert-butyl methyl ether. The extracted diterpenes are then purified and esterified with palmitic acid to produce this compound.
Chemical Reactions Analysis
Types of Reactions: Cafestol palmitate primarily undergoes esterification and hydrolysis reactions.
Common Reagents and Conditions:
Esterification: Strong acid catalysts (e.g., sulfuric acid) and heat under reflux conditions.
Hydrolysis: Base-catalyzed hydrolysis using potassium hydroxide (KOH) in a water bath.
Major Products Formed:
Esterification: this compound is formed from cafestol and palmitic acid.
Hydrolysis: Cafestol and palmitic acid are the products of hydrolysis.
Scientific Research Applications
Medicine: It exhibits anticancer properties by inhibiting the proliferation and migration of cancer cells.
Biology: It has anti-inflammatory effects and can modulate immune responses.
Chemistry: It is used in the development of functional foods and nutraceuticals due to its cholesterol-lowering properties.
Industry: It is utilized in the production of coffee-related products and supplements.
Mechanism of Action
Cafestol palmitate exerts its effects through multiple pathways:
Molecular Targets: It interacts with nuclear hormone receptors such as the farnesoid X receptor (FXR) and pregnane X receptor (PXR).
Pathways Involved: It modulates gene expression related to lipid metabolism and bile acid homeostasis, leading to changes in serum lipid levels.
Comparison with Similar Compounds
Kahweol: Another diterpene found in coffee beans with similar bioactivities.
16-O-Methylcafestol: A methylated derivative of cafestol found in robusta coffee.
Does this cover everything you were looking for, or is there something specific you'd like to know more about?
Properties
IUPAC Name |
[(1S,4S,12S,13R,16R,17R)-17-hydroxy-12-methyl-8-oxapentacyclo[14.2.1.01,13.04,12.05,9]nonadeca-5(9),6-dien-17-yl]methyl hexadecanoate | |
---|---|---|
Source | PubChem | |
URL | https://pubchem.ncbi.nlm.nih.gov | |
Description | Data deposited in or computed by PubChem | |
InChI |
InChI=1S/C36H58O4/c1-3-4-5-6-7-8-9-10-11-12-13-14-15-16-33(37)40-27-36(38)26-35-23-19-30-29-21-24-39-31(29)20-22-34(30,2)32(35)18-17-28(36)25-35/h21,24,28,30,32,38H,3-20,22-23,25-27H2,1-2H3/t28-,30-,32+,34-,35+,36+/m1/s1 | |
Source | PubChem | |
URL | https://pubchem.ncbi.nlm.nih.gov | |
Description | Data deposited in or computed by PubChem | |
InChI Key |
DGPMHJKMSANHDM-SMDVAALKSA-N | |
Source | PubChem | |
URL | https://pubchem.ncbi.nlm.nih.gov | |
Description | Data deposited in or computed by PubChem | |
Canonical SMILES |
CCCCCCCCCCCCCCCC(=O)OCC1(CC23CCC4C5=C(CCC4(C2CCC1C3)C)OC=C5)O | |
Source | PubChem | |
URL | https://pubchem.ncbi.nlm.nih.gov | |
Description | Data deposited in or computed by PubChem | |
Isomeric SMILES |
CCCCCCCCCCCCCCCC(=O)OC[C@]1(C[C@@]23CC[C@@H]4C5=C(CC[C@]4([C@@H]2CC[C@@H]1C3)C)OC=C5)O | |
Source | PubChem | |
URL | https://pubchem.ncbi.nlm.nih.gov | |
Description | Data deposited in or computed by PubChem | |
Molecular Formula |
C36H58O4 | |
Source | PubChem | |
URL | https://pubchem.ncbi.nlm.nih.gov | |
Description | Data deposited in or computed by PubChem | |
Molecular Weight |
554.8 g/mol | |
Source | PubChem | |
URL | https://pubchem.ncbi.nlm.nih.gov | |
Description | Data deposited in or computed by PubChem | |
CAS No. |
81760-46-5 | |
Record name | Cafestol palmitate | |
Source | ChemIDplus | |
URL | https://pubchem.ncbi.nlm.nih.gov/substance/?source=chemidplus&sourceid=0081760465 | |
Description | ChemIDplus is a free, web search system that provides access to the structure and nomenclature authority files used for the identification of chemical substances cited in National Library of Medicine (NLM) databases, including the TOXNET system. | |
Record name | CAFESTOL PALMITATE | |
Source | FDA Global Substance Registration System (GSRS) | |
URL | https://gsrs.ncats.nih.gov/ginas/app/beta/substances/P8W9M3T86Y | |
Description | The FDA Global Substance Registration System (GSRS) enables the efficient and accurate exchange of information on what substances are in regulated products. Instead of relying on names, which vary across regulatory domains, countries, and regions, the GSRS knowledge base makes it possible for substances to be defined by standardized, scientific descriptions. | |
Explanation | Unless otherwise noted, the contents of the FDA website (www.fda.gov), both text and graphics, are not copyrighted. They are in the public domain and may be republished, reprinted and otherwise used freely by anyone without the need to obtain permission from FDA. Credit to the U.S. Food and Drug Administration as the source is appreciated but not required. | |
Retrosynthesis Analysis
AI-Powered Synthesis Planning: Our tool employs the Template_relevance Pistachio, Template_relevance Bkms_metabolic, Template_relevance Pistachio_ringbreaker, Template_relevance Reaxys, Template_relevance Reaxys_biocatalysis model, leveraging a vast database of chemical reactions to predict feasible synthetic routes.
One-Step Synthesis Focus: Specifically designed for one-step synthesis, it provides concise and direct routes for your target compounds, streamlining the synthesis process.
Accurate Predictions: Utilizing the extensive PISTACHIO, BKMS_METABOLIC, PISTACHIO_RINGBREAKER, REAXYS, REAXYS_BIOCATALYSIS database, our tool offers high-accuracy predictions, reflecting the latest in chemical research and data.
Strategy Settings
Precursor scoring | Relevance Heuristic |
---|---|
Min. plausibility | 0.01 |
Model | Template_relevance |
Template Set | Pistachio/Bkms_metabolic/Pistachio_ringbreaker/Reaxys/Reaxys_biocatalysis |
Top-N result to add to graph | 6 |
Feasible Synthetic Routes
Disclaimer and Information on In-Vitro Research Products
Please be aware that all articles and product information presented on BenchChem are intended solely for informational purposes. The products available for purchase on BenchChem are specifically designed for in-vitro studies, which are conducted outside of living organisms. In-vitro studies, derived from the Latin term "in glass," involve experiments performed in controlled laboratory settings using cells or tissues. It is important to note that these products are not categorized as medicines or drugs, and they have not received approval from the FDA for the prevention, treatment, or cure of any medical condition, ailment, or disease. We must emphasize that any form of bodily introduction of these products into humans or animals is strictly prohibited by law. It is essential to adhere to these guidelines to ensure compliance with legal and ethical standards in research and experimentation.