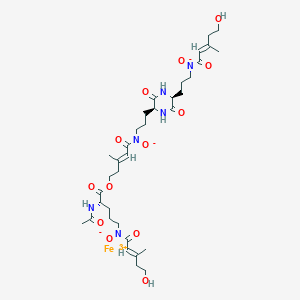
Coprogen
Overview
Description
Coprogen is a hydroxamate-type siderophore produced by fungi to facilitate iron acquisition under iron-deficient conditions. Structurally, it consists of three N-acyl-N-hydroxyornithine (AMHO) units linked via ester bonds, forming a linear trihydroxamate scaffold . Biosynthetically, this compound is synthesized by nonribosomal peptide synthetase (NRPS) gene clusters, particularly mrsidA and mrsidD, as demonstrated in Metarhizium robertsii and Penicillium species . Its production is upregulated during iron starvation, with carbon and nitrogen availability significantly influencing yield in submerged cultures .
Functionally, this compound chelates Fe(III) with high affinity (logβ ~ 30), enabling fungi to solubilize and internalize iron from the environment . For example, hydrolysates of this compound enhance iron uptake in both Strategy I (e.g., cucumber) and Strategy II (e.g., maize) plants, improving iron bioavailability in crops . Additionally, Penicillium nalgiovense-fermented foods, such as certain cheeses and sausages, contain high this compound levels, suggesting its use in iron-fortified functional foods .
Preparation Methods
Biosynthetic Preparation of Coprogen in Fungi
This compound is primarily biosynthesized by fungi such as Neurospora crassa and Metarhizium robertsii. Its biosynthesis is encoded by specific gene clusters that orchestrate the enzymatic assembly of this compound and related siderophores.
Genetic and Enzymatic Basis
- The biosynthesis involves non-ribosomal peptide synthetases (NRPS), particularly the mrsidD gene in Metarhizium robertsii, which is responsible for producing both this compound and dimerumic acid siderophores.
- Gene clusters related to iron deficiency regulate the expression of biosynthetic enzymes, as iron scarcity triggers siderophore production.
- Genetic deletion studies (knockouts of mrsidA and mrsidD) abolish this compound production, confirming their essential role.
- Mannosylated derivatives of this compound have been identified, indicating glycosyltransferase activity outside the main biosynthetic gene clusters, suggesting potential for structural diversity in this compound compounds.
Proposed Biosynthetic Pathway
- This compound biosynthesis starts from precursor molecules such as N5-hydroxy-N5-acetylornithine (AMHO) units.
- Three AMHO units are enzymatically linked to form the this compound backbone.
- Dimerumic acid, a related siderophore, consists of two AMHO units forming a diketopiperazine ring and may act as a precursor or degradation product of this compound.
- Mannosylation occurs post-assembly, enhancing biological function and structural diversity.
Optimization of this compound Production in Culture
The preparation of this compound on a preparative scale typically involves fungal fermentation under optimized culture conditions to maximize yield.
Culture Medium Composition
- The synthetic medium for Neurospora crassa includes glucose, aspartate, K2HPO4, MgSO4, CaCl2, ZnSO4, and biotin.
- Key variables affecting this compound production are glucose concentration, aspartate concentration (nitrogen source), and initial pH.
Effects of Medium Components and Culture Conditions
Parameter | Range Tested | Optimal Value for this compound Production | Notes |
---|---|---|---|
Glucose | 10–60 g/L | >40 g/L (optimal at 40 g/L in shake flasks) | Higher glucose increased biomass but too high caused viscosity issues |
Aspartate | 5–50 g/L | 21 g/L | Nitrogen source critical for siderophore biosynthesis |
Initial pH | 4.0–9.0 | 7.2 | Optimal pH for enzyme activity and fungal growth |
Temperature | 28 °C | 28 °C | Standard fungal growth temperature |
Aeration & Stirring | 2 L/min aeration, 200 rpm stirring (bioreactor) | Maintained for scale-up fermentation | Ensures oxygen supply and homogeneity |
Fermentation Setup
- Shake flask cultures: 100 mL medium inoculated with fresh mycelia, incubated at 28 °C and 250 rpm for up to 10 days.
- Bioreactor fermentation: 2 L volume, inoculated with pre-cultured fungi, aerated at 2 L/min, stirred at 200 rpm, pH stabilized at 7.2, cultivated for 7 days.
Production Kinetics
- This compound production increases with time, peaking around 7 days.
- Dry cell mass correlates with glucose concentration, influencing this compound yield.
- Response surface methodology confirmed the interaction of pH and aspartate concentration significantly affects yields.
Isolation and Purification of this compound
Following biosynthesis, this compound is isolated and purified using chromatographic techniques.
Sample Preparation
- Culture broth is filtered to remove fungal biomass.
- Filtrates are supplemented with FeCl3 to form ferri-siderophore complexes, which are more stable and easier to detect.
Chromatographic Purification
- High-performance liquid chromatography (HPLC) with reverse-phase columns (e.g., Spherisorb ODS2, 250×4.6 mm, 5 μm) is used.
- Gradient elution with water/acetonitrile mixtures is employed.
- Detection wavelengths typically include 220 nm and 435 nm to monitor siderophore peaks.
- Semi-preparative HPLC is used for isolating this compound and related compounds in milligram quantities.
Summary Table of Preparation Parameters and Outcomes
Step | Method/Condition | Outcome/Notes |
---|---|---|
Biosynthesis Organism | Neurospora crassa, Metarhizium robertsii | Efficient natural producers of this compound |
Genetic Control | NRPS genes (mrsidD, mrsidA) | Essential for this compound biosynthesis |
Medium Composition | Glucose (40 g/L), Aspartate (21 g/L), pH 7.2 | Optimized for maximum this compound yield |
Culture Conditions | 28 °C, 7 days, aerobic fermentation | High biomass and siderophore production |
Isolation Technique | Filtration + FeCl3 complexation + HPLC | Purified this compound suitable for structural and functional studies |
Analytical Methods | NMR, HR-ESI-MS, UV-Vis detection | Confirm structure and purity |
Research Findings and Implications
- The biosynthetic gene clusters and enzymatic pathways of this compound have been elucidated, enabling genetic manipulation for enhanced production or structural modification.
- Optimization of culture media and conditions significantly improves this compound yield, facilitating scalable production.
- Mannosylated this compound derivatives suggest potential for novel siderophore variants with improved biological activities.
- The purification methods ensure high-purity this compound suitable for biochemical and structural studies, as well as potential pharmaceutical applications.
Chemical Reactions Analysis
Types of Reactions: Coprogen undergoes various chemical reactions, including:
Oxidation: this compound can be oxidized to form its ferric complex, which is the form in which it binds iron (III) ions.
Reduction: The ferric complex of this compound can be reduced back to its ferrous form under certain conditions.
Substitution: this compound can participate in substitution reactions where the hydroxamate groups are replaced by other functional groups.
Common Reagents and Conditions:
Oxidation: Hydrogen peroxide or other oxidizing agents can be used to oxidize this compound.
Reduction: Reducing agents such as sodium dithionite can be used to reduce the ferric complex of this compound.
Substitution: Various nucleophiles can be used in substitution reactions to modify the hydroxamate groups of this compound.
Major Products Formed:
Ferric this compound Complex: Formed through oxidation and subsequent binding of iron (III) ions.
Modified this compound Derivatives: Formed through substitution reactions.
Scientific Research Applications
Microbial Iron Acquisition
Coprogen plays a crucial role in iron acquisition for various microorganisms. It is produced by fungi such as Talaromyces marneffei, which enhances its survival in iron-limited environments. Research has shown that mutants of T. marneffei with enhanced this compound production can significantly reduce the iron levels available to competing pathogens, demonstrating its potential as an ecological competitive strategy .
Medical Applications
Iron Chelation Therapy
This compound and its derivatives have emerged as potential iron chelators in medical applications. For instance, this compound B has been shown to reduce labile iron pools in hepatocellular carcinoma (Huh7) cells without exhibiting toxicity. This property suggests its utility in treating conditions related to iron overload, such as hemochromatosis and certain types of cancer .
Antimicrobial Properties
The antimicrobial activity of this compound B has been documented against various pathogens, including Candida albicans and Escherichia coli. Studies indicate that this compound B inhibits the growth of these organisms in a dose-dependent manner, suggesting its potential as an alternative therapeutic agent in treating infections caused by resistant strains .
Agricultural Applications
This compound's role in promoting plant growth through enhanced nutrient availability is being explored. By improving the bioavailability of iron in soils deficient in this nutrient, this compound can enhance plant health and yield. This application is particularly relevant in regions with high soil pH where iron availability is limited .
Case Study 1: this compound Production Enhancement
A study focused on genetically modifying Talaromyces marneffei to enhance this compound production demonstrated that deletion of the SreA gene led to increased extracellular siderophore levels. The resulting strain produced this compound B, which was characterized using HPLC-MS and NMR techniques .
Key Findings:
- Enhanced Production : The modified strain showed significantly higher this compound levels.
- Iron Binding : this compound B effectively reduced iron levels in cultured cells.
- Antimicrobial Activity : Demonstrated effectiveness against pathogenic bacteria.
Case Study 2: this compound as an Antimicrobial Agent
Research investigating the antimicrobial properties of this compound B revealed its effectiveness against several bacterial strains. The study assessed growth inhibition through various concentrations of this compound B, confirming its potential as a natural antimicrobial agent .
Key Findings:
- Inhibition Rates : Significant growth inhibition observed at specific concentrations.
- Mechanism of Action : Likely involves iron sequestration from pathogens.
Table 1: Comparison of this compound Production in Various Fungi
Table 2: Antimicrobial Activity of this compound B
Mechanism of Action
Coprogen exerts its effects by binding iron (III) ions with high specificity and affinity. The hydroxamate groups in this compound form stable complexes with iron (III) ions, facilitating their transport into microbial cells. Once inside the cell, the iron is released and utilized in various metabolic processes, including DNA replication, amino acid biosynthesis, and the citric acid cycle. The molecular targets of this compound include iron (III)-hydroxamate-binding proteins such as FhuD in Escherichia coli .
Comparison with Similar Compounds
Coprogen belongs to a family of structurally related fungal siderophores. Below, we compare its properties with dimerum acid, neocoprogens, and this compound B.
Key Observations :
- Dimerum acid is structurally simpler, with studies suggesting it arises from this compound degradation via hydrolysis or enzymatic cleavage . However, genetic evidence in M. robertsii indicates shared biosynthesis pathways, implying dimerum acid may also act as a precursor .
- Neocoprogens (I and II) differ in N-acyl substitutions, reducing their iron-binding efficiency compared to this compound. For instance, neothis compound II inhibits this compound uptake by only 21% at equimolar concentrations due to reduced receptor affinity .
- This compound B , a methylated variant, lacks the terminal acetyl group of this compound, altering its solubility and iron-release kinetics .
Functional and Ecological Roles
Key Observations :
- This compound is the most versatile, with applications in both microbial and plant systems. Its hydrolysates (containing dimerum acid and fusarinines) are 2–3 times more effective than intact this compound in promoting plant iron absorption .
- Dimerum acid’s role remains debated: while Penicillium chrysogenum produces it as a natural siderophore, Metarhizium studies suggest it is a breakdown product .
- This compound B’s methylation enhances stability in acidic environments, making it preferable in industrial fungal fermentations .
Genetic and Production Insights
Biological Activity
Coprogen is a siderophore produced by various fungi, particularly within the genus Aspergillus and Talaromyces. It plays a crucial role in iron acquisition, which is vital for microbial growth and pathogenicity. This article explores the biological activities of this compound, focusing on its mechanisms of action, antimicrobial properties, and potential applications in medical science.
Iron Scavenging : this compound functions primarily as an iron-chelating agent. It binds to ferric iron (Fe³⁺) with high affinity, thereby facilitating iron uptake in environments where this essential nutrient is limited. This property is particularly important for pathogenic fungi that require iron for virulence.
Biosynthesis Pathway : Recent studies have elucidated the biosynthetic pathways of this compound in fungi. For instance, genetic analyses in Metarhizium robertsii revealed specific gene clusters responsible for this compound synthesis, indicating a complex regulatory mechanism influenced by environmental iron levels .
2. Antimicrobial Properties
This compound exhibits antimicrobial activity against various pathogens. Research has demonstrated that this compound B, a derivative of this compound, effectively inhibits the growth of Candida albicans and Escherichia coli . The compound's effectiveness varies with concentration, indicating a dose-dependent response in its antimicrobial activity.
Table 1: Antimicrobial Activity of this compound B
Pathogen | Minimum Inhibitory Concentration (MIC) | Reference |
---|---|---|
Candida albicans | 32 µg/mL | |
Escherichia coli | 64 µg/mL |
3. Case Studies
Several case studies highlight the significance of this compound in clinical and environmental contexts:
- Case Study on Iron Chelation : In a study involving hepatocellular carcinoma (Huh7) cells, this compound B was shown to reduce labile iron pools without exhibiting cytotoxic effects, suggesting its potential as an iron chelator in cancer therapy .
- Environmental Impact : Research indicates that this compound plays a role in microbial competition for iron in soil ecosystems. By outcompeting other microorganisms for iron, fungi producing this compound can establish dominance in nutrient-poor environments .
4. Research Findings
Recent research has focused on the genetic engineering of fungi to enhance this compound production. For example, deletion of the sreA gene in Talaromyces marneffei led to increased this compound production, highlighting genetic manipulation as a viable strategy to boost siderophore yields for therapeutic applications .
Q & A
Basic Research Questions
Q. What methodologies are recommended for characterizing Coprogen in fungal cultures?
this compound can be isolated via solvent extraction (e.g., ethyl acetate) from fungal supernatants, followed by purification using column chromatography. Structural characterization requires UV-Vis spectroscopy (absorption peaks at 315 nm and 450 nm for iron-bound and free forms, respectively), high-resolution mass spectrometry (HRMS), and nuclear magnetic resonance (NMR) spectroscopy. Iron-binding capacity is confirmed through chrome azurol S (CAS) assays . Table 1: Key Characterization Techniques
Method | Purpose | Parameters/Notes |
---|---|---|
CAS assay | Siderophore activity confirmation | Blue-to-orange color shift indicates iron chelation |
HRMS | Molecular weight determination | Positive ion mode; m/z ~780 ([M+H]+) |
NMR (1H, 13C) | Structural elucidation | Hydroxamate signals at δ 8.1 ppm (1H) |
Q. How can researchers standardize fungal culturing conditions to optimize this compound production?
Use iron-limited media (e.g., SM medium) to induce siderophore biosynthesis. Control variables include pH (5.5–6.5), temperature (25–28°C), and agitation speed (120–150 rpm). Quantify yield via HPLC with a C18 column and UV detection at 315 nm. Reference Hesseltine et al. (1952) for baseline protocols .
Q. What assays are suitable for evaluating this compound’s role in fungal iron acquisition?
Competitive growth assays under iron-limiting conditions: Co-culture this compound-producing fungi with non-producing strains or bacteria. Measure growth rates via OD600 or colony-forming units (CFUs). Supplementation with 10–100 µM FeCl3 can validate iron dependency .
Advanced Research Questions
Q. How can molecular dynamics simulations clarify this compound’s interaction with TonB-dependent transporters in Gram-negative bacteria?
Use homology modeling (e.g., SWISS-MODEL) to predict the FhuE transporter structure. Perform docking studies (AutoDock Vina) to map this compound binding sites. Validate predictions with site-directed mutagenesis of key residues (e.g., His210, Arg345) and measure uptake efficiency via 55Fe radiolabeling assays .
Q. What experimental strategies address discrepancies in this compound’s reported phytotoxic activity?
Conflicting data (e.g., weak activity in Alternaria cassia vs. strong effects in other species) may arise from differences in plant models, application methods (foliar vs. root exposure), or dosage (µg/mL vs. mg/mL). Conduct dose-response curves (0.1–100 µg/mL) on standardized plant systems (e.g., Arabidopsis thaliana) and quantify oxidative stress markers (e.g., malondialdehyde) .
Q. How can researchers design co-culture experiments to study this compound-mediated microbial competition?
Use dual-chamber systems (e.g., Transwell plates) to separate fungi and bacteria while allowing metabolite exchange. Monitor iron availability via ferrozine assays and gene expression (qPCR) of bacterial iron-regulated genes (e.g., fhuE). Include controls with iron supplementation or this compound-deficient fungal mutants .
Q. What approaches mitigate this compound’s instability in aqueous solutions during long-term experiments?
Stabilize this compound using cryopreservation (-80°C in 10% glycerol) or lyophilization. For in vitro assays, add antioxidants (e.g., 1 mM ascorbate) and avoid prolonged light exposure. Validate stability via HPLC at 0, 24, and 48-hour timepoints .
Q. How can genetic manipulation of Pilobolus spp. enhance this compound yield for functional studies?
Knock out regulatory genes (e.g., sidA for siderophore biosynthesis) via CRISPR-Cas9 to create hyper-producing strains. Overexpress efflux pumps (e.g., mfs1) to improve secretion. Quantify transcriptional changes using RNA-seq and correlate with siderophore titers .
Q. What ecological study designs evaluate this compound’s impact on soil microbiome dynamics?
Conduct microcosm experiments with sterilized soil inoculated with defined microbial communities. Apply 1–10 µM this compound and track microbial diversity via 16S/ITS sequencing. Measure iron bioavailability through sequential extraction (e.g., Tessier method) .
Q. Methodological Considerations for Data Rigor
- Reproducibility : Document detailed protocols for this compound extraction, purification, and bioassays in Supporting Information (per Beilstein Journal of Organic Chemistry guidelines) .
- Data Contradictions : Apply triangulation (e.g., combine CAS assays, gene expression, and radiolabeling) to validate siderophore functionality .
- Ethical Compliance : For plant studies, adhere to institutional biosafety guidelines for phytotoxin handling .
Properties
CAS No. |
31418-71-0 |
---|---|
Molecular Formula |
C35H53FeN6O13 |
Molecular Weight |
821.7 g/mol |
IUPAC Name |
[(E)-5-[3-[(2S,5S)-5-[3-[[(E)-5-hydroxy-3-methylpent-2-enoyl]-oxidoamino]propyl]-3,6-dioxopiperazin-2-yl]propyl-oxidoamino]-3-methyl-5-oxopent-3-enyl] (2S)-2-acetamido-5-[[(E)-5-hydroxy-3-methylpent-2-enoyl]-oxidoamino]pentanoate;iron(3+) |
InChI |
InChI=1S/C35H53N6O13.Fe/c1-23(11-17-42)20-30(45)39(51)14-5-8-27-33(48)38-28(34(49)37-27)9-6-15-40(52)32(47)22-25(3)13-19-54-35(50)29(36-26(4)44)10-7-16-41(53)31(46)21-24(2)12-18-43;/h20-22,27-29,42-43H,5-19H2,1-4H3,(H,36,44)(H,37,49)(H,38,48);/q-3;+3/b23-20+,24-21+,25-22+;/t27-,28-,29-;/m0./s1 |
InChI Key |
FQIVLXIUJLOKPL-DWZMLRRXSA-N |
SMILES |
CC(=CC(=O)N(CCCC1C(=O)NC(C(=O)N1)CCCN(C(=O)C=C(C)CCOC(=O)C(CCCN(C(=O)C=C(C)CCO)[O-])NC(=O)C)[O-])[O-])CCO.[Fe+3] |
Isomeric SMILES |
C/C(=C\C(=O)N(CCC[C@H]1C(=O)N[C@H](C(=O)N1)CCCN(C(=O)/C=C(\C)/CCOC(=O)[C@H](CCCN(C(=O)/C=C(\C)/CCO)[O-])NC(=O)C)[O-])[O-])/CCO.[Fe+3] |
Canonical SMILES |
CC(=CC(=O)N(CCCC1C(=O)NC(C(=O)N1)CCCN(C(=O)C=C(C)CCOC(=O)C(CCCN(C(=O)C=C(C)CCO)[O-])NC(=O)C)[O-])[O-])CCO.[Fe+3] |
Key on ui other cas no. |
31418-71-0 |
Synonyms |
coprogen |
Origin of Product |
United States |
Retrosynthesis Analysis
AI-Powered Synthesis Planning: Our tool employs the Template_relevance Pistachio, Template_relevance Bkms_metabolic, Template_relevance Pistachio_ringbreaker, Template_relevance Reaxys, Template_relevance Reaxys_biocatalysis model, leveraging a vast database of chemical reactions to predict feasible synthetic routes.
One-Step Synthesis Focus: Specifically designed for one-step synthesis, it provides concise and direct routes for your target compounds, streamlining the synthesis process.
Accurate Predictions: Utilizing the extensive PISTACHIO, BKMS_METABOLIC, PISTACHIO_RINGBREAKER, REAXYS, REAXYS_BIOCATALYSIS database, our tool offers high-accuracy predictions, reflecting the latest in chemical research and data.
Strategy Settings
Precursor scoring | Relevance Heuristic |
---|---|
Min. plausibility | 0.01 |
Model | Template_relevance |
Template Set | Pistachio/Bkms_metabolic/Pistachio_ringbreaker/Reaxys/Reaxys_biocatalysis |
Top-N result to add to graph | 6 |
Feasible Synthetic Routes
Disclaimer and Information on In-Vitro Research Products
Please be aware that all articles and product information presented on BenchChem are intended solely for informational purposes. The products available for purchase on BenchChem are specifically designed for in-vitro studies, which are conducted outside of living organisms. In-vitro studies, derived from the Latin term "in glass," involve experiments performed in controlled laboratory settings using cells or tissues. It is important to note that these products are not categorized as medicines or drugs, and they have not received approval from the FDA for the prevention, treatment, or cure of any medical condition, ailment, or disease. We must emphasize that any form of bodily introduction of these products into humans or animals is strictly prohibited by law. It is essential to adhere to these guidelines to ensure compliance with legal and ethical standards in research and experimentation.