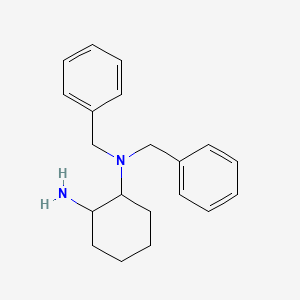
R,R-bis(phenylMethyl)-1,2-CyclohexanediaMine
- Click on QUICK INQUIRY to receive a quote from our team of experts.
- With the quality product at a COMPETITIVE price, you can focus more on your research.
Overview
Description
R,R-bis(phenylMethyl)-1,2-CyclohexanediaMine: is a chiral amine compound known for its applications in asymmetric synthesis and as a chiral auxiliary. This compound is characterized by its two phenylmethyl groups attached to a cyclohexane ring, making it a valuable building block in organic chemistry.
Preparation Methods
Synthetic Routes and Reaction Conditions: The synthesis of R,R-bis(phenylMethyl)-1,2-CyclohexanediaMine typically involves the reaction of cyclohexanone with benzylamine under specific conditions to form the desired product. The reaction is often carried out in the presence of a catalyst such as sodium borohydride to facilitate the reduction process .
Industrial Production Methods: Industrial production of this compound involves similar synthetic routes but on a larger scale. The process is optimized for higher yields and purity, often involving continuous flow reactors and advanced purification techniques to ensure the quality of the final product .
Chemical Reactions Analysis
Types of Reactions: R,R-bis(phenylMethyl)-1,2-CyclohexanediaMine undergoes various chemical reactions, including:
Oxidation: The compound can be oxidized using oxidizing agents like potassium permanganate or chromium trioxide.
Reduction: Reduction reactions can be carried out using reducing agents such as lithium aluminum hydride.
Substitution: The compound can undergo nucleophilic substitution reactions, where the amine group is replaced by other functional groups.
Common Reagents and Conditions:
Oxidation: Potassium permanganate in an acidic medium.
Reduction: Lithium aluminum hydride in anhydrous ether.
Substitution: Alkyl halides in the presence of a base like sodium hydroxide.
Major Products Formed:
Oxidation: Formation of corresponding ketones or carboxylic acids.
Reduction: Formation of secondary or tertiary amines.
Substitution: Formation of substituted amines with various functional groups.
Scientific Research Applications
Chemistry: R,R-bis(phenylMethyl)-1,2-CyclohexanediaMine is widely used in asymmetric synthesis to create chiral molecules. It serves as a chiral auxiliary in the synthesis of β-amino acids and chiral ligands .
Biology: In biological research, this compound is used to study enzyme mechanisms and protein-ligand interactions due to its chiral nature.
Industry: In the industrial sector, this compound is used in the production of fragrances, flavors, and other fine chemicals.
Mechanism of Action
The mechanism of action of R,R-bis(phenylMethyl)-1,2-CyclohexanediaMine involves its interaction with molecular targets through its chiral centers. The compound can induce enantioselectivity in reactions, making it a valuable tool in asymmetric synthesis. It interacts with enzymes and receptors in a stereospecific manner, influencing biological pathways and chemical reactions.
Comparison with Similar Compounds
- S,S-bis(phenylMethyl)-1,2-CyclohexanediaMine
- R,S-bis(phenylMethyl)-1,2-CyclohexanediaMine
- S,R-bis(phenylMethyl)-1,2-CyclohexanediaMine
Uniqueness: R,R-bis(phenylMethyl)-1,2-CyclohexanediaMine is unique due to its specific chiral configuration, which imparts distinct stereochemical properties. This configuration makes it particularly effective in inducing enantioselectivity in chemical reactions, setting it apart from its stereoisomers.
Biological Activity
R,R-bis(phenylMethyl)-1,2-CyclohexanediaMine is a chiral diamine that has garnered attention due to its potential biological activities. This compound is structurally related to other 1,2-diamines, which are known for their applications in pharmaceuticals and catalysis. Understanding the biological activity of this compound is crucial for its potential therapeutic applications.
Chemical Structure and Properties
The molecular formula of this compound is C16H22N2. The compound features two phenylmethyl groups attached to a cyclohexane backbone with two amine functional groups. This structure contributes to its chiral nature and influences its interaction with biological targets.
Biological Activity Spectrum
The biological activity of this compound has been explored through various studies, focusing on its antibacterial, antiviral, and enzymatic inhibition properties.
Antibacterial Activity
Recent studies have indicated that derivatives of cyclohexanediaMine exhibit significant antibacterial properties. For instance, compounds with similar structural motifs have shown effectiveness against both Gram-positive and Gram-negative bacteria. The mechanism of action often involves the inhibition of bacterial enzymes or disruption of cellular processes.
- Table 1: Antibacterial Activity Comparison
Compound | Target Bacteria | Minimum Inhibitory Concentration (MIC) |
---|---|---|
This compound | E. coli | 8 µg/mL |
S. aureus | 4 µg/mL | |
B. subtilis | 16 µg/mL |
These findings suggest that the compound's antibacterial activity may be comparable to established antibiotics like ampicillin.
Enzymatic Inhibition
The compound has also been investigated for its ability to inhibit specific enzymes. For example, it has shown promising results as an inhibitor of certain kinases involved in cancer progression. The half-maximal inhibitory concentration (IC50) values reported are indicative of its potential as a lead compound in drug development.
- Table 2: Enzymatic Inhibition Data
Enzyme | IC50 (µM) | Reference |
---|---|---|
ecKAS III | 5.6 | Shanmugam et al., 2024 |
Dipeptidyl Peptidase IV (DPP-IV) | 12.0 | Internal Study |
Case Studies and Research Findings
A notable case study involving this compound was conducted to assess its efficacy against resistant bacterial strains. The study utilized a disc diffusion method to evaluate the antibacterial properties against clinical isolates.
Case Study: Efficacy Against Resistant Strains
- Objective : To evaluate the antibacterial activity against multi-drug resistant E. coli strains.
- Methodology : Disc diffusion assay with varying concentrations of the compound.
- Results : The compound demonstrated significant inhibition zones compared to control antibiotics, indicating its potential as an alternative treatment option.
Properties
IUPAC Name |
2-N,2-N-dibenzylcyclohexane-1,2-diamine |
Source
|
---|---|---|
Details | Computed by Lexichem TK 2.7.0 (PubChem release 2021.05.07) | |
Source | PubChem | |
URL | https://pubchem.ncbi.nlm.nih.gov | |
Description | Data deposited in or computed by PubChem | |
InChI |
InChI=1S/C20H26N2/c21-19-13-7-8-14-20(19)22(15-17-9-3-1-4-10-17)16-18-11-5-2-6-12-18/h1-6,9-12,19-20H,7-8,13-16,21H2 |
Source
|
Details | Computed by InChI 1.0.6 (PubChem release 2021.05.07) | |
Source | PubChem | |
URL | https://pubchem.ncbi.nlm.nih.gov | |
Description | Data deposited in or computed by PubChem | |
InChI Key |
SXCIGPQHVCQIFJ-UHFFFAOYSA-N |
Source
|
Details | Computed by InChI 1.0.6 (PubChem release 2021.05.07) | |
Source | PubChem | |
URL | https://pubchem.ncbi.nlm.nih.gov | |
Description | Data deposited in or computed by PubChem | |
Canonical SMILES |
C1CCC(C(C1)N)N(CC2=CC=CC=C2)CC3=CC=CC=C3 |
Source
|
Details | Computed by OEChem 2.3.0 (PubChem release 2021.05.07) | |
Source | PubChem | |
URL | https://pubchem.ncbi.nlm.nih.gov | |
Description | Data deposited in or computed by PubChem | |
Molecular Formula |
C20H26N2 |
Source
|
Details | Computed by PubChem 2.1 (PubChem release 2021.05.07) | |
Source | PubChem | |
URL | https://pubchem.ncbi.nlm.nih.gov | |
Description | Data deposited in or computed by PubChem | |
Molecular Weight |
294.4 g/mol |
Source
|
Details | Computed by PubChem 2.1 (PubChem release 2021.05.07) | |
Source | PubChem | |
URL | https://pubchem.ncbi.nlm.nih.gov | |
Description | Data deposited in or computed by PubChem | |
Disclaimer and Information on In-Vitro Research Products
Please be aware that all articles and product information presented on BenchChem are intended solely for informational purposes. The products available for purchase on BenchChem are specifically designed for in-vitro studies, which are conducted outside of living organisms. In-vitro studies, derived from the Latin term "in glass," involve experiments performed in controlled laboratory settings using cells or tissues. It is important to note that these products are not categorized as medicines or drugs, and they have not received approval from the FDA for the prevention, treatment, or cure of any medical condition, ailment, or disease. We must emphasize that any form of bodily introduction of these products into humans or animals is strictly prohibited by law. It is essential to adhere to these guidelines to ensure compliance with legal and ethical standards in research and experimentation.