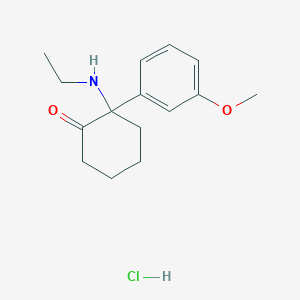
Methoxetamine hydrochloride
Overview
Description
Methoxetamine (hydrochloride) is a synthetic dissociative anesthetic belonging to the arylcyclohexylamine class. It was developed as a designer drug and is known for its dissociative and hallucinogenic effects. Methoxetamine (hydrochloride) acts primarily as an N-methyl-D-aspartate (NMDA) receptor antagonist, similar to other compounds in its class such as ketamine and phencyclidine .
Mechanism of Action
Target of Action
Methoxetamine hydrochloride primarily targets the N-methyl-D-aspartate (NMDA) receptors . These receptors are a type of ionotropic glutamate receptor and play a crucial role in controlling synaptic plasticity and memory function .
Mode of Action
This compound acts mainly as an NMDA receptor antagonist . This means it binds to these receptors and inhibits their activity. The inhibition of NMDA receptors by this compound leads to a decrease in neuronal excitability, which results in its dissociative effects .
Biochemical Pathways
For instance, NMDA receptor antagonism has been linked to increased activity of the mammalian target of rapamycin (mTOR) pathway , which plays a key role in cell growth, cell proliferation, protein synthesis, and synaptic plasticity .
Pharmacokinetics
It is known that the compound has a high affinity for nmda receptors , suggesting that it may be readily absorbed and distributed in the body.
Result of Action
The molecular and cellular effects of this compound’s action primarily involve the inhibition of NMDA receptor activity. This can lead to a range of effects, including altered perception, cognitive disruption, and potential neurotoxicity . At the cellular level, this compound can induce cytotoxicity in certain cell types .
Biochemical Analysis
Biochemical Properties
Methoxetamine hydrochloride interacts with several enzymes, proteins, and other biomolecules. It acts mainly as an NMDA receptor antagonist . It was often believed to possess opioid properties due to its structural similarity to 3-HO-PCP, but this assumption is not supported by data, which shows insignificant affinity for the μ-opioid receptor by the compound .
Cellular Effects
This compound has been found to have significant effects on various types of cells and cellular processes. For instance, it has been found to induce cytotoxicity in H9c2 cells, a cell line derived from rat heart tissue . This cytotoxicity is possibly related to the regulation of the expression and function of P21 protein (Cdc42/Rac)-activated kinase 1 (PAK-1) .
Molecular Mechanism
The mechanism of action of this compound is primarily through its antagonistic effect on the NMDA receptor . It has also been found to act as a serotonin reuptake inhibitor . It shows little or no effect on the reuptake of dopamine and norepinephrine .
Temporal Effects in Laboratory Settings
It has been associated with hospitalizations from high and/or combined consumption in the US and UK . Acute reversible cerebellar toxicity has been documented in three cases of hospital admission due to MXE overdose, lasting for between one and four days after exposure .
Metabolic Pathways
This compound is involved in several metabolic pathways. It undergoes O-demethylation, hydroxylation, N-deethylation, dehydrogenation, and reduction .
Preparation Methods
The synthesis of methoxetamine (hydrochloride) involves several steps starting from commercially available compounds. One common synthetic route includes the following steps :
Formation of an aromatic nitrile: This involves the reaction of an aromatic compound with a nitrile group.
Grignard reaction: The aromatic nitrile undergoes a Grignard reaction to form an intermediate compound.
Bromination: The intermediate compound is then brominated.
Imine formation: The brominated compound reacts with an appropriate amine to form an imine.
Cyclization: The imine undergoes cyclization upon heating to form methoxetamine.
Industrial production methods typically involve optimizing these steps to achieve high yields and purity. The reaction conditions, such as temperature, solvents, and catalysts, are carefully controlled to ensure the desired product is obtained.
Chemical Reactions Analysis
Methoxetamine (hydrochloride) undergoes various chemical reactions, including :
Oxidation: Methoxetamine can be oxidized to form various metabolites. Common oxidizing agents include potassium permanganate and chromium trioxide.
Reduction: Reduction reactions can convert methoxetamine to its corresponding amine derivatives. Reducing agents such as lithium aluminum hydride are often used.
Substitution: Methoxetamine can undergo substitution reactions where functional groups are replaced by other groups. For example, halogenation can introduce halogen atoms into the molecule.
The major products formed from these reactions depend on the specific reagents and conditions used. For instance, oxidation typically results in the formation of hydroxylated metabolites.
Scientific Research Applications
Methoxetamine (hydrochloride) has several scientific research applications :
Chemistry: It is used as a reference standard in analytical chemistry for the identification and quantification of similar compounds.
Biology: Methoxetamine is studied for its effects on the central nervous system, particularly its interaction with NMDA receptors.
Medicine: Research is ongoing to explore its potential therapeutic applications, such as its antidepressant effects.
Industry: Methoxetamine is used in the development of new psychoactive substances and as a tool for studying the pharmacology of dissociative anesthetics.
Comparison with Similar Compounds
Methoxetamine (hydrochloride) is often compared to other dissociative anesthetics such as ketamine and phencyclidine . Here are some key points of comparison:
Ketamine: Both methoxetamine and ketamine are NMDA receptor antagonists, but methoxetamine is designed to have a longer duration of action and fewer side effects related to bladder toxicity.
Phencyclidine (PCP): Methoxetamine shares structural similarities with phencyclidine but is considered to have a safer profile and less potential for abuse.
Similar compounds include:
- Ketamine
- Phencyclidine (PCP)
- N-ethylnorketamine (NENK)
- 2-MeO-N-ethylketamine (2-MeO-NEK)
- 4-MeO-N-ethylketamine (4-MeO-NEK)
Methoxetamine’s unique properties, such as its specific receptor interactions and reduced side effects, make it a valuable compound for research and potential therapeutic applications.
Properties
IUPAC Name |
2-(ethylamino)-2-(3-methoxyphenyl)cyclohexan-1-one;hydrochloride | |
---|---|---|
Source | PubChem | |
URL | https://pubchem.ncbi.nlm.nih.gov | |
Description | Data deposited in or computed by PubChem | |
InChI |
InChI=1S/C15H21NO2.ClH/c1-3-16-15(10-5-4-9-14(15)17)12-7-6-8-13(11-12)18-2;/h6-8,11,16H,3-5,9-10H2,1-2H3;1H | |
Source | PubChem | |
URL | https://pubchem.ncbi.nlm.nih.gov | |
Description | Data deposited in or computed by PubChem | |
InChI Key |
FJNRBMKLTGCSRN-UHFFFAOYSA-N | |
Source | PubChem | |
URL | https://pubchem.ncbi.nlm.nih.gov | |
Description | Data deposited in or computed by PubChem | |
Canonical SMILES |
CCNC1(CCCCC1=O)C2=CC(=CC=C2)OC.Cl | |
Source | PubChem | |
URL | https://pubchem.ncbi.nlm.nih.gov | |
Description | Data deposited in or computed by PubChem | |
Molecular Formula |
C15H22ClNO2 | |
Source | PubChem | |
URL | https://pubchem.ncbi.nlm.nih.gov | |
Description | Data deposited in or computed by PubChem | |
Molecular Weight |
283.79 g/mol | |
Source | PubChem | |
URL | https://pubchem.ncbi.nlm.nih.gov | |
Description | Data deposited in or computed by PubChem | |
CAS No. |
1239908-48-5 | |
Record name | Cyclohexanone, 2-(ethylamino)-2-(3-methoxyphenyl)-, hydrochloride (1:1) | |
Source | CAS Common Chemistry | |
URL | https://commonchemistry.cas.org/detail?cas_rn=1239908-48-5 | |
Description | CAS Common Chemistry is an open community resource for accessing chemical information. Nearly 500,000 chemical substances from CAS REGISTRY cover areas of community interest, including common and frequently regulated chemicals, and those relevant to high school and undergraduate chemistry classes. This chemical information, curated by our expert scientists, is provided in alignment with our mission as a division of the American Chemical Society. | |
Explanation | The data from CAS Common Chemistry is provided under a CC-BY-NC 4.0 license, unless otherwise stated. | |
Record name | Methoxetamine hydrochloride | |
Source | ChemIDplus | |
URL | https://pubchem.ncbi.nlm.nih.gov/substance/?source=chemidplus&sourceid=1239908485 | |
Description | ChemIDplus is a free, web search system that provides access to the structure and nomenclature authority files used for the identification of chemical substances cited in National Library of Medicine (NLM) databases, including the TOXNET system. | |
Record name | METHOXETAMINE HYDROCHLORIDE | |
Source | FDA Global Substance Registration System (GSRS) | |
URL | https://gsrs.ncats.nih.gov/ginas/app/beta/substances/DKO8XUO3NY | |
Description | The FDA Global Substance Registration System (GSRS) enables the efficient and accurate exchange of information on what substances are in regulated products. Instead of relying on names, which vary across regulatory domains, countries, and regions, the GSRS knowledge base makes it possible for substances to be defined by standardized, scientific descriptions. | |
Explanation | Unless otherwise noted, the contents of the FDA website (www.fda.gov), both text and graphics, are not copyrighted. They are in the public domain and may be republished, reprinted and otherwise used freely by anyone without the need to obtain permission from FDA. Credit to the U.S. Food and Drug Administration as the source is appreciated but not required. | |
Retrosynthesis Analysis
AI-Powered Synthesis Planning: Our tool employs the Template_relevance Pistachio, Template_relevance Bkms_metabolic, Template_relevance Pistachio_ringbreaker, Template_relevance Reaxys, Template_relevance Reaxys_biocatalysis model, leveraging a vast database of chemical reactions to predict feasible synthetic routes.
One-Step Synthesis Focus: Specifically designed for one-step synthesis, it provides concise and direct routes for your target compounds, streamlining the synthesis process.
Accurate Predictions: Utilizing the extensive PISTACHIO, BKMS_METABOLIC, PISTACHIO_RINGBREAKER, REAXYS, REAXYS_BIOCATALYSIS database, our tool offers high-accuracy predictions, reflecting the latest in chemical research and data.
Strategy Settings
Precursor scoring | Relevance Heuristic |
---|---|
Min. plausibility | 0.01 |
Model | Template_relevance |
Template Set | Pistachio/Bkms_metabolic/Pistachio_ringbreaker/Reaxys/Reaxys_biocatalysis |
Top-N result to add to graph | 6 |
Feasible Synthetic Routes
Q1: What is the significance of the crystallographic data obtained for methoxetamine hydrochloride?
A1: The study successfully determined the crystal structure of this compound using X-ray powder diffraction. This technique revealed key structural information, including:
Disclaimer and Information on In-Vitro Research Products
Please be aware that all articles and product information presented on BenchChem are intended solely for informational purposes. The products available for purchase on BenchChem are specifically designed for in-vitro studies, which are conducted outside of living organisms. In-vitro studies, derived from the Latin term "in glass," involve experiments performed in controlled laboratory settings using cells or tissues. It is important to note that these products are not categorized as medicines or drugs, and they have not received approval from the FDA for the prevention, treatment, or cure of any medical condition, ailment, or disease. We must emphasize that any form of bodily introduction of these products into humans or animals is strictly prohibited by law. It is essential to adhere to these guidelines to ensure compliance with legal and ethical standards in research and experimentation.