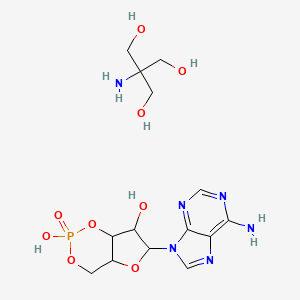
Adenosine 3'
- Click on QUICK INQUIRY to receive a quote from our team of experts.
- With the quality product at a COMPETITIVE price, you can focus more on your research.
Overview
Description
Adenosine 3 is a compound that belongs to the family of adenosine derivatives. Adenosine itself is a nucleoside composed of adenine and ribose, playing a crucial role in various biochemical processes, including energy transfer and signal transduction. Adenosine 3, specifically, is known for its interaction with the A3 adenosine receptor, which is involved in numerous physiological and pathological processes.
Preparation Methods
Synthetic Routes and Reaction Conditions: The synthesis of Adenosine 3 typically involves the modification of adenosine through various chemical reactions. One common method includes the use of selective alkylation or acylation reactions to introduce specific functional groups at the desired positions on the adenosine molecule. The reaction conditions often involve the use of organic solvents, catalysts, and controlled temperatures to achieve high yields and purity.
Industrial Production Methods: In an industrial setting, the production of Adenosine 3 may involve large-scale chemical synthesis using automated reactors and continuous flow systems. The process is optimized for efficiency, cost-effectiveness, and environmental sustainability. Key steps include the purification of intermediates and the final product using techniques such as chromatography and crystallization.
Chemical Reactions Analysis
Types of Reactions: Adenosine 3 undergoes various chemical reactions, including:
Oxidation: Involves the addition of oxygen or the removal of hydrogen, often using oxidizing agents like hydrogen peroxide or potassium permanganate.
Reduction: Involves the addition of hydrogen or the removal of oxygen, typically using reducing agents such as sodium borohydride or lithium aluminum hydride.
Substitution: Involves the replacement of one functional group with another, often using nucleophilic or electrophilic reagents.
Common Reagents and Conditions:
Oxidation: Hydrogen peroxide, potassium permanganate, and other oxidizing agents under acidic or basic conditions.
Reduction: Sodium borohydride, lithium aluminum hydride, and other reducing agents under controlled temperatures.
Substitution: Nucleophiles like halides, amines, or thiols, and electrophiles like alkyl halides or acyl chlorides.
Major Products: The major products formed from these reactions depend on the specific reagents and conditions used. For example, oxidation may yield adenosine derivatives with additional oxygen-containing functional groups, while reduction may produce more saturated compounds.
Scientific Research Applications
Adenosine 3 has a wide range of scientific research applications, including:
Chemistry: Used as a building block for the synthesis of more complex molecules and as a reagent in various chemical reactions.
Biology: Studied for its role in cellular signaling and energy transfer, particularly in the context of the A3 adenosine receptor.
Medicine: Investigated for its potential therapeutic effects in conditions such as inflammation, cancer, and cardiovascular diseases. It is also used in diagnostic procedures and as a pharmacological tool to study receptor functions.
Industry: Utilized in the production of pharmaceuticals, biochemical assays, and as a component in various industrial processes.
Mechanism of Action
Adenosine 3 exerts its effects primarily through its interaction with the A3 adenosine receptor, a G-protein-coupled receptor. Upon binding to the receptor, Adenosine 3 activates intracellular signaling pathways that involve the inhibition of adenylate cyclase, reduction of cyclic adenosine monophosphate levels, and modulation of ion channel activity. These actions lead to various physiological responses, including anti-inflammatory, anti-cancer, and cardioprotective effects.
Comparison with Similar Compounds
Adenosine: The parent compound, involved in energy transfer and signal transduction.
Adenosine 5: Another derivative with distinct receptor interactions and physiological effects.
Adenosine 2: Known for its role in vasodilation and anti-inflammatory effects.
Uniqueness: Adenosine 3 is unique due to its high affinity and selectivity for the A3 adenosine receptor, making it a valuable tool in both research and therapeutic applications. Its distinct chemical structure allows for specific interactions with the receptor, leading to targeted physiological responses that are not observed with other adenosine derivatives.
Properties
Molecular Formula |
C14H23N6O9P |
---|---|
Molecular Weight |
450.34 g/mol |
IUPAC Name |
2-amino-2-(hydroxymethyl)propane-1,3-diol;6-(6-aminopurin-9-yl)-2-hydroxy-2-oxo-4a,6,7,7a-tetrahydro-4H-furo[3,2-d][1,3,2]dioxaphosphinin-7-ol |
InChI |
InChI=1S/C10H12N5O6P.C4H11NO3/c11-8-5-9(13-2-12-8)15(3-14-5)10-6(16)7-4(20-10)1-19-22(17,18)21-7;5-4(1-6,2-7)3-8/h2-4,6-7,10,16H,1H2,(H,17,18)(H2,11,12,13);6-8H,1-3,5H2 |
InChI Key |
CLDDYJFNXTUHIN-UHFFFAOYSA-N |
Canonical SMILES |
C1C2C(C(C(O2)N3C=NC4=C(N=CN=C43)N)O)OP(=O)(O1)O.C(C(CO)(CO)N)O |
Origin of Product |
United States |
Disclaimer and Information on In-Vitro Research Products
Please be aware that all articles and product information presented on BenchChem are intended solely for informational purposes. The products available for purchase on BenchChem are specifically designed for in-vitro studies, which are conducted outside of living organisms. In-vitro studies, derived from the Latin term "in glass," involve experiments performed in controlled laboratory settings using cells or tissues. It is important to note that these products are not categorized as medicines or drugs, and they have not received approval from the FDA for the prevention, treatment, or cure of any medical condition, ailment, or disease. We must emphasize that any form of bodily introduction of these products into humans or animals is strictly prohibited by law. It is essential to adhere to these guidelines to ensure compliance with legal and ethical standards in research and experimentation.