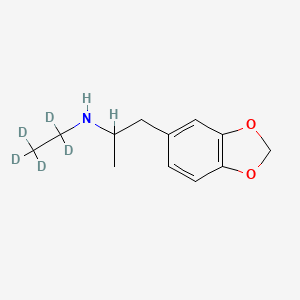
rac-MDEA-D5
Overview
Description
Rac-MDEA-D5, also known as N-methyl-N-[2-(2-methoxyethoxy)ethyl]benzylamine, is an important chemical compound used in a variety of scientific research applications. It is a derivative of the amine group and is used in a variety of biochemical and physiological experiments.
Scientific Research Applications
Research Methodologies and Technologies
Raman-Activated Cell Sorting for Compound Analysis
Raman-activated cell sorting (RACS) is a technology that combines dielectrophoresis for single-cell trap and release with Raman spectroscopy for cell identification. This method, being label-free and information-rich, could potentially be used for studying the effects of compounds like "rac-MDEA-D5" on single cells, enabling detailed analysis of chemical interactions at a cellular level (Zhang et al., 2015).
Genetic Polymorphisms and Drug Efficacy
The study of genetic polymorphisms, such as those in the MDR1 gene, can influence the efficacy of drug treatments. Understanding how genetic variations affect the action of drugs can be crucial for personalized medicine. This approach could be relevant for evaluating the effectiveness of "this compound" in different populations, considering genetic backgrounds (Muralidharan et al., 2015).
Mechanism of Action
Pharmacokinetics
These properties generally include a balance between lipophilicity and hydrophilicity, which can impact a compound’s bioavailability .
Action Environment
Environmental factors can influence the action, efficacy, and stability of a compound like rac-MDEA-D5. For example, the presence of certain acids has been found to influence the makeup flow rate of methyldiethanolamine (MDEA), a compound related to this compound . Additionally, factors such as temperature, pH, and the presence of other substances can potentially impact the action and stability of this compound.
properties
IUPAC Name |
1-(1,3-benzodioxol-5-yl)-N-(1,1,2,2,2-pentadeuterioethyl)propan-2-amine | |
---|---|---|
Source | PubChem | |
URL | https://pubchem.ncbi.nlm.nih.gov | |
Description | Data deposited in or computed by PubChem | |
InChI |
InChI=1S/C12H17NO2/c1-3-13-9(2)6-10-4-5-11-12(7-10)15-8-14-11/h4-5,7,9,13H,3,6,8H2,1-2H3/i1D3,3D2 | |
Source | PubChem | |
URL | https://pubchem.ncbi.nlm.nih.gov | |
Description | Data deposited in or computed by PubChem | |
InChI Key |
PVXVWWANJIWJOO-WNWXXORZSA-N | |
Source | PubChem | |
URL | https://pubchem.ncbi.nlm.nih.gov | |
Description | Data deposited in or computed by PubChem | |
Canonical SMILES |
CCNC(C)CC1=CC2=C(C=C1)OCO2 | |
Source | PubChem | |
URL | https://pubchem.ncbi.nlm.nih.gov | |
Description | Data deposited in or computed by PubChem | |
Isomeric SMILES |
[2H]C([2H])([2H])C([2H])([2H])NC(C)CC1=CC2=C(C=C1)OCO2 | |
Source | PubChem | |
URL | https://pubchem.ncbi.nlm.nih.gov | |
Description | Data deposited in or computed by PubChem | |
Molecular Formula |
C12H17NO2 | |
Source | PubChem | |
URL | https://pubchem.ncbi.nlm.nih.gov | |
Description | Data deposited in or computed by PubChem | |
Molecular Weight |
212.30 g/mol | |
Source | PubChem | |
URL | https://pubchem.ncbi.nlm.nih.gov | |
Description | Data deposited in or computed by PubChem | |
CAS RN |
160227-43-0 | |
Record name | 160227-43-0 | |
Source | European Chemicals Agency (ECHA) | |
URL | https://echa.europa.eu/information-on-chemicals | |
Description | The European Chemicals Agency (ECHA) is an agency of the European Union which is the driving force among regulatory authorities in implementing the EU's groundbreaking chemicals legislation for the benefit of human health and the environment as well as for innovation and competitiveness. | |
Explanation | Use of the information, documents and data from the ECHA website is subject to the terms and conditions of this Legal Notice, and subject to other binding limitations provided for under applicable law, the information, documents and data made available on the ECHA website may be reproduced, distributed and/or used, totally or in part, for non-commercial purposes provided that ECHA is acknowledged as the source: "Source: European Chemicals Agency, http://echa.europa.eu/". Such acknowledgement must be included in each copy of the material. ECHA permits and encourages organisations and individuals to create links to the ECHA website under the following cumulative conditions: Links can only be made to webpages that provide a link to the Legal Notice page. | |
Retrosynthesis Analysis
AI-Powered Synthesis Planning: Our tool employs the Template_relevance Pistachio, Template_relevance Bkms_metabolic, Template_relevance Pistachio_ringbreaker, Template_relevance Reaxys, Template_relevance Reaxys_biocatalysis model, leveraging a vast database of chemical reactions to predict feasible synthetic routes.
One-Step Synthesis Focus: Specifically designed for one-step synthesis, it provides concise and direct routes for your target compounds, streamlining the synthesis process.
Accurate Predictions: Utilizing the extensive PISTACHIO, BKMS_METABOLIC, PISTACHIO_RINGBREAKER, REAXYS, REAXYS_BIOCATALYSIS database, our tool offers high-accuracy predictions, reflecting the latest in chemical research and data.
Strategy Settings
Precursor scoring | Relevance Heuristic |
---|---|
Min. plausibility | 0.01 |
Model | Template_relevance |
Template Set | Pistachio/Bkms_metabolic/Pistachio_ringbreaker/Reaxys/Reaxys_biocatalysis |
Top-N result to add to graph | 6 |
Feasible Synthetic Routes
Disclaimer and Information on In-Vitro Research Products
Please be aware that all articles and product information presented on BenchChem are intended solely for informational purposes. The products available for purchase on BenchChem are specifically designed for in-vitro studies, which are conducted outside of living organisms. In-vitro studies, derived from the Latin term "in glass," involve experiments performed in controlled laboratory settings using cells or tissues. It is important to note that these products are not categorized as medicines or drugs, and they have not received approval from the FDA for the prevention, treatment, or cure of any medical condition, ailment, or disease. We must emphasize that any form of bodily introduction of these products into humans or animals is strictly prohibited by law. It is essential to adhere to these guidelines to ensure compliance with legal and ethical standards in research and experimentation.