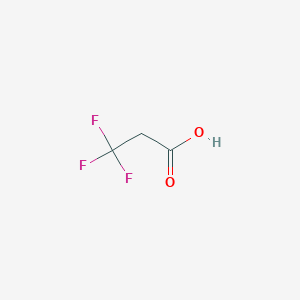
3,3,3-Trifluoropropionic acid
Overview
Description
3,3,3-Trifluoropropionic acid (TriFPA, CAS 2516-99-6) is a short-chain fluorinated carboxylic acid with the molecular formula C₃H₃F₃O₂ and a molecular weight of 128.05 g/mol . It is a colorless liquid with a boiling point of 145°C, density of 1.45 g/mL, and a pKa of 1.70 . Its strong acidity and trifluoromethyl group make it a valuable intermediate in organic synthesis, particularly for pharmaceuticals, agrochemicals, and fluorinated polymers . TriFPA is also notable for its environmental relevance, as it is a byproduct of advanced reduction processes for per- and polyfluoroalkyl substances (PFAS) and can undergo microbial defluorination .
Scientific Research Applications
Synthesis of 3,3,3-Trifluoropropionic Acid
TFPA can be synthesized through various methods, including the reaction of trifluoroacetic anhydride with propionic acid derivatives. This process allows for the production of TFPA as an intermediate for various chemical reactions and applications in pharmaceuticals and agrochemicals .
Drug Development
TFPA serves as a crucial intermediate in the synthesis of several pharmaceutical compounds. Its unique trifluoromethyl group enhances the biological activity of certain drugs by improving their metabolic stability and bioavailability. For instance, TFPA derivatives have been investigated for their potential as anti-inflammatory and anticancer agents .
Case Study: Synthesis of Anticancer Agents
A study demonstrated the use of TFPA in synthesizing novel anticancer agents that exhibited enhanced efficacy against specific cancer cell lines. The incorporation of the trifluoromethyl group was shown to significantly improve the compounds' potency compared to their non-fluorinated analogs .
Biodegradation Studies
Recent research has highlighted the role of TFPA in environmental science, particularly concerning its biodegradability. Studies indicate that TFPA can undergo aerobic defluorination by microbial communities, suggesting its potential for bioremediation strategies aimed at mitigating fluorinated pollutants in wastewater treatment systems .
Case Study: Aerobic Defluorination
In one study, activated sludge communities were shown to effectively defluorinate TFPA, achieving nearly complete removal within a short time frame. This finding underscores the compound's susceptibility to microbial degradation and its implications for environmental safety .
Use as a Solvent and Reagent
TFPA is utilized as a solvent and reagent in various industrial chemical processes due to its unique properties that facilitate reactions involving nucleophiles and electrophiles. Its high boiling point makes it suitable for high-temperature reactions in organic synthesis .
Case Study: Polymer Production
The application of TFPA in producing fluorinated polymers has been explored extensively. Its incorporation into polymer matrices enhances thermal stability and chemical resistance, making it valuable in manufacturing specialized materials used in electronics and coatings .
Mechanism of Action
The mechanism by which 3,3,3-Trifluoropropionic acid exerts its effects is primarily through its strong electron-withdrawing trifluoromethyl group. This group influences the reactivity and stability of the compound, making it a potent intermediate in various chemical reactions. The molecular targets and pathways involved depend on the specific application, such as enzyme inhibition in biological studies or catalytic activity in industrial processes .
Comparison with Similar Compounds
Comparison with Similar Fluorinated Carboxylic Acids
Acidity and Reactivity
The trifluoromethyl group significantly enhances acidity compared to non-fluorinated analogs. Table 1 compares TriFPA with other short-chain fluorinated and non-fluorinated carboxylic acids:
*Estimated based on structural trends .
TriFPA’s intermediate acidity enables unique reactivity in electrochemical reactions. For example, under Hofer–Moest conditions, TriFPA yields Kolbe dimerization products (42%) and low C–O bond formation (e.g., ethers), unlike non-fluorinated propionic acid, which favors decarboxylative coupling .
Biodegradation and Environmental Fate
TriFPA exhibits superior biodegradability compared to persistent PFAS like perfluorobutane sulfonic acid (PFBS) . In contrast, longer-chain fluorinated acids (e.g., 5,5,5-trifluoropentanoic acid) show <20% defluorination under similar conditions .
Structural and Physical Properties
The trifluoromethyl group at the β-position distinguishes TriFPA from other fluorinated propionic acids. For example:
Biological Activity
3,3,3-Trifluoropropionic acid (TFPA), with the chemical formula CHFO and CAS number 2516-99-6, is a fluorinated carboxylic acid that has garnered attention for its unique biological properties and potential applications in various fields, including medicinal chemistry and environmental science. This article aims to provide a comprehensive overview of the biological activity of TFPA, including its mechanisms of action, biodegradation pathways, and relevant case studies.
- Molecular Weight : 128.05 g/mol
- IUPAC Name : 3,3,3-trifluoropropanoic acid
- Synonyms : Trifluoromethylacetic acid, TFPA
- Structure :
Mechanisms of Biological Activity
TFPA exhibits several biological activities that are primarily attributed to its fluorinated structure. The presence of fluorine atoms can significantly alter the reactivity and interaction of the compound with biological systems.
1. Antimicrobial Activity
Research has indicated that TFPA possesses antimicrobial properties. A study demonstrated that TFPA can inhibit the growth of certain bacterial strains by disrupting their metabolic processes. The exact mechanism is believed to involve interference with enzyme functions critical for bacterial survival .
2. Biodegradation and Defluorination
TFPA is subject to biodegradation processes mediated by specific microorganisms. Notably, Pseudomonas putida F1 has been shown to catalyze the defluorination of TFPA at significant rates. This process involves the conversion of TFPA into less harmful products through enzymatic pathways that utilize toluene dioxygenase . The defluorination rates observed were approximately 2,100 nmol/h per mg of cellular protein, indicating a highly efficient microbial degradation pathway .
Case Study 1: Microbial Defluorination
A detailed examination of microbial defluorination highlighted the role of Pseudomonas putida in degrading TFPA under aerobic conditions. The study tracked the release of fluoride ions during the degradation process, demonstrating a direct correlation between microbial activity and fluoride release. Over time, significant color changes in the medium indicated metabolic activity linked to defluorination .
Case Study 2: Synthesis and Applications
TFPA has been utilized as an intermediate in synthesizing various chemical compounds, including pharmaceuticals and agrochemicals. Its unique properties make it a valuable building block in developing fluorinated derivatives that exhibit enhanced biological activity. For instance, it serves as a precursor for producing trifluoropropionyl chloride, which is used in synthesizing functional materials .
Data Tables
Property | Value |
---|---|
Molecular Formula | CHFO |
Molecular Weight | 128.05 g/mol |
CAS Number | 2516-99-6 |
IUPAC Name | 3,3,3-trifluoropropanoic acid |
Antimicrobial Activity | Yes |
Biodegradation Rate | 2100 nmol/h/mg protein |
Q & A
Basic Research Questions
Q. What are the primary synthetic routes for 3,3,3-trifluoropropionic acid, and how do reaction conditions influence yield?
The synthesis of this compound has been optimized using UV light photocatalysis, achieving high yields under controlled wavelength and solvent conditions (e.g., acetonitrile as a solvent). Electrochemical methods, such as electrodecarboxylative etherification, employ Li₂CO₃ as a base and methanol/pyridine mixtures, with yields dependent on current density and reaction time . Contaminants like residual fluorinated byproducts must be monitored via ¹⁹F NMR or LC-MS to ensure purity .
Q. How do the physicochemical properties of this compound impact its experimental applications?
The compound’s pKa (~1.5–2.0) and high polarity make it a strong acid, suitable for use as a catalyst or mobile-phase additive in LC/MS. Its low boiling point (~247°C) and density (1.5 g/cm³) necessitate careful handling in distillation or high-temperature reactions . The trifluoromethyl group enhances electron-withdrawing effects, influencing reactivity in nucleophilic substitutions .
Q. What safety protocols are critical when handling this compound in laboratory settings?
As a corrosive liquid (UN 3265), it requires acid-resistant gloves (e.g., nitrile), goggles, and ventilation. Immediate decontamination with water is essential for skin/eye exposure. Waste must be neutralized with alkaline solutions (e.g., sodium bicarbonate) before disposal . Storage in sealed containers at ≤25°C prevents degradation .
Q. Which analytical techniques are most effective for quantifying this compound in environmental samples?
LC-MS with reverse-phase columns (C18) and acidic mobile phases (e.g., 0.1% formic acid) provides high sensitivity for environmental matrices. Solid-phase extraction (SPE) using hydrophilic-lipophilic balance (HLB) cartridges improves detection limits in water samples. ¹⁹F NMR is preferred for structural confirmation and purity assessment .
Advanced Research Questions
Q. How do structural modifications (e.g., aryl substituents) alter the reactivity and stability of this compound derivatives?
Substituting the α-carbon with electron-withdrawing groups (e.g., nitro or halogens) increases acidity and stabilizes intermediates in coupling reactions. For example, 2-(3-nitrophenyl)-3,3,3-trifluoropropionic acid exhibits enhanced electrophilicity in amidation reactions, while bulky substituents (e.g., biphenyl) reduce solubility, requiring polar aprotic solvents like DMF .
Q. What mechanistic insights explain the role of this compound in electrochemical decarboxylation reactions?
In electrodecarboxylative etherification, the acid acts as a carboxylate source under anodic oxidation. Li₂CO₃ facilitates deprotonation, generating a trifluoromethyl radical intermediate, which couples with nucleophiles (e.g., benzotriazole). The reaction’s Faradaic efficiency (e.g., 62% yield at 500 mA/30 min) depends on electrode material (Pt) and solvent polarity .
Q. How can researchers resolve contradictions in reported environmental persistence data for this compound?
Discrepancies in half-life data (e.g., soil vs. aquatic systems) may arise from variable microbial degradation rates. Standardized OECD 301B biodegradation tests under controlled pH and temperature are recommended. Confounding factors like co-pollutants (e.g., perfluoroalkyl substances) should be analyzed via high-resolution mass spectrometry .
Q. What strategies optimize the scalability of this compound synthesis while minimizing fluorinated waste?
Continuous-flow reactors improve heat management and reduce side reactions in photochemical synthesis. Catalytic hydrogenation (e.g., Pd/C at 30 bar H₂) minimizes byproducts in downstream derivatization. Solvent recovery systems (e.g., fractional distillation) are critical for recycling methanol/pyridine mixtures .
Preparation Methods
Oxidation of 3,3,3-Trifluoropropanal
The most extensively documented method involves oxidizing 3,3,3-trifluoropropanal using hydrogen peroxide (H₂O₂) under acidic conditions . This approach prioritizes atom economy and minimizes inorganic waste compared to traditional oxidants like potassium permanganate.
Reaction Mechanism and Conditions
The oxidation proceeds via acid-catalyzed nucleophilic attack of H₂O₂ on the aldehyde group, forming the corresponding carboxylic acid. Key parameters include:
-
Temperature : 60–100°C
-
H₂O₂-to-Aldehyde Molar Ratio : 1.1:1 to 2.5:1
-
Catalyst Loading : 2–10 wt% relative to aldehyde
Sulfuric acid, phospho-tungstic acid, and mesoporous sulfonic acid are effective catalysts . For example, a 1.5:1 H₂O₂-to-aldehyde ratio with 5% mesoporous sulfonic acid at 100°C for 8 hours achieves an 85% yield .
Catalyst Performance Comparison
Table 1 summarizes yields under varying catalysts and conditions:
Catalyst | Temperature (°C) | Time (h) | Yield (%) |
---|---|---|---|
Mesoporous sulfonic acid | 100 | 8 | 85.0 |
Sulfuric acid | 90 | 10 | 84.3 |
Phospho-tungstic acid | 100 | 9 | 82.4 |
Nitric acid | 90 | 8 | 82.1 |
Higher temperatures (>90°C) and prolonged reaction times (>8 hours) generally improve conversion but risk side reactions, such as over-oxidation or decomposition .
Environmental and Practical Considerations
Using H₂O₂ eliminates salt-laden wastewater associated with permanganate-based methods . Post-reaction purification involves diethyl ether extraction and vacuum distillation, yielding TFPA with ≥98.5% purity . Nuclear magnetic resonance (NMR) data confirm structural integrity:
Hydrolysis of Ester Precursors
Alternative routes involve hydrolyzing ethyl 3,3,3-trifluoropropionate or related esters. A 2022 study demonstrates sulfuric acid-mediated hydrolysis of ethyl 3,3,3-trifluoro-2-(trifluoromethyl)propanoate .
Hydrolysis Conditions
-
Reagent : 50% H₂SO₄
-
Temperature : 90°C
-
Duration : 20 hours
This method achieves a 61% yield, with extraction using diethyl ether and distillation refining the product . While less efficient than direct oxidation, it avoids handling gaseous aldehydes, simplifying scale-up.
Acid Chloride Synthesis
TFPA derivatives like 3,3,3-trifluoropropionyl chloride are accessible via phosphorus pentachloride (PCl₅) treatment :
This intermediate is valuable for synthesizing amides and esters used in drug discovery .
Comparative Analysis of Methods
Table 2 contrasts the two primary methods:
Parameter | Oxidation of Aldehyde | Ester Hydrolysis |
---|---|---|
Starting Material Cost | Moderate | Low |
Yield | 82–85% | 61% |
Byproducts | H₂O | Alcohols |
Scalability | High | Moderate |
Environmental Impact | Low | Moderate |
The aldehyde oxidation route is preferred for industrial applications due to superior yields and greener chemistry .
Emerging Approaches and Innovations
Recent patents explore solid acid catalysts (e.g., sulfated zirconia) to enhance recyclability . Additionally, flow chemistry systems are being tested to improve heat management during exothermic oxidation steps .
Properties
IUPAC Name |
3,3,3-trifluoropropanoic acid | |
---|---|---|
Source | PubChem | |
URL | https://pubchem.ncbi.nlm.nih.gov | |
Description | Data deposited in or computed by PubChem | |
InChI |
InChI=1S/C3H3F3O2/c4-3(5,6)1-2(7)8/h1H2,(H,7,8) | |
Source | PubChem | |
URL | https://pubchem.ncbi.nlm.nih.gov | |
Description | Data deposited in or computed by PubChem | |
InChI Key |
KSNKQSPJFRQSEI-UHFFFAOYSA-N | |
Source | PubChem | |
URL | https://pubchem.ncbi.nlm.nih.gov | |
Description | Data deposited in or computed by PubChem | |
Canonical SMILES |
C(C(=O)O)C(F)(F)F | |
Source | PubChem | |
URL | https://pubchem.ncbi.nlm.nih.gov | |
Description | Data deposited in or computed by PubChem | |
Molecular Formula |
C3H3F3O2 | |
Source | PubChem | |
URL | https://pubchem.ncbi.nlm.nih.gov | |
Description | Data deposited in or computed by PubChem | |
DSSTOX Substance ID |
DTXSID70380740 | |
Record name | 3,3,3-Trifluoropropionic acid | |
Source | EPA DSSTox | |
URL | https://comptox.epa.gov/dashboard/DTXSID70380740 | |
Description | DSSTox provides a high quality public chemistry resource for supporting improved predictive toxicology. | |
Molecular Weight |
128.05 g/mol | |
Source | PubChem | |
URL | https://pubchem.ncbi.nlm.nih.gov | |
Description | Data deposited in or computed by PubChem | |
CAS No. |
2516-99-6 | |
Record name | 3,3,3-Trifluoropropionic acid | |
Source | EPA DSSTox | |
URL | https://comptox.epa.gov/dashboard/DTXSID70380740 | |
Description | DSSTox provides a high quality public chemistry resource for supporting improved predictive toxicology. | |
Record name | 3,3,3-Trifluoropropionic acid | |
Source | European Chemicals Agency (ECHA) | |
URL | https://echa.europa.eu/information-on-chemicals | |
Description | The European Chemicals Agency (ECHA) is an agency of the European Union which is the driving force among regulatory authorities in implementing the EU's groundbreaking chemicals legislation for the benefit of human health and the environment as well as for innovation and competitiveness. | |
Explanation | Use of the information, documents and data from the ECHA website is subject to the terms and conditions of this Legal Notice, and subject to other binding limitations provided for under applicable law, the information, documents and data made available on the ECHA website may be reproduced, distributed and/or used, totally or in part, for non-commercial purposes provided that ECHA is acknowledged as the source: "Source: European Chemicals Agency, http://echa.europa.eu/". Such acknowledgement must be included in each copy of the material. ECHA permits and encourages organisations and individuals to create links to the ECHA website under the following cumulative conditions: Links can only be made to webpages that provide a link to the Legal Notice page. | |
Synthesis routes and methods
Procedure details
Retrosynthesis Analysis
AI-Powered Synthesis Planning: Our tool employs the Template_relevance Pistachio, Template_relevance Bkms_metabolic, Template_relevance Pistachio_ringbreaker, Template_relevance Reaxys, Template_relevance Reaxys_biocatalysis model, leveraging a vast database of chemical reactions to predict feasible synthetic routes.
One-Step Synthesis Focus: Specifically designed for one-step synthesis, it provides concise and direct routes for your target compounds, streamlining the synthesis process.
Accurate Predictions: Utilizing the extensive PISTACHIO, BKMS_METABOLIC, PISTACHIO_RINGBREAKER, REAXYS, REAXYS_BIOCATALYSIS database, our tool offers high-accuracy predictions, reflecting the latest in chemical research and data.
Strategy Settings
Precursor scoring | Relevance Heuristic |
---|---|
Min. plausibility | 0.01 |
Model | Template_relevance |
Template Set | Pistachio/Bkms_metabolic/Pistachio_ringbreaker/Reaxys/Reaxys_biocatalysis |
Top-N result to add to graph | 6 |
Feasible Synthetic Routes
Disclaimer and Information on In-Vitro Research Products
Please be aware that all articles and product information presented on BenchChem are intended solely for informational purposes. The products available for purchase on BenchChem are specifically designed for in-vitro studies, which are conducted outside of living organisms. In-vitro studies, derived from the Latin term "in glass," involve experiments performed in controlled laboratory settings using cells or tissues. It is important to note that these products are not categorized as medicines or drugs, and they have not received approval from the FDA for the prevention, treatment, or cure of any medical condition, ailment, or disease. We must emphasize that any form of bodily introduction of these products into humans or animals is strictly prohibited by law. It is essential to adhere to these guidelines to ensure compliance with legal and ethical standards in research and experimentation.