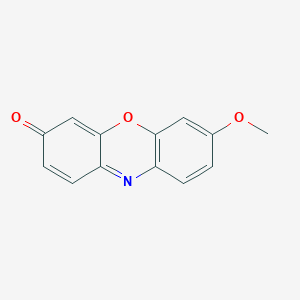
7-Methoxy-3H-phenoxazin-3-one
Overview
Description
Methoxyresorufin: (CAS Number: 5725-89-3) is a fluorogenic substrate primarily used for assessing the activity of cytochrome P450 (CYP) isoforms, specifically CYP1A1 and CYP1A2 . It belongs to the class of phenoxazinone derivatives and exhibits fluorescence upon enzymatic metabolism.
Mechanism of Action
Target of Action
The primary targets of Methoxyresorufin are nitroreductase and cytochrome P450 (CYP1A) . Nitroreductase is an enzyme produced by Escherichia coli that plays a crucial role in the reduction of nitro compounds . Cytochrome P450 is a family of enzymes involved in drug metabolism and bioactivation .
Mode of Action
Methoxyresorufin interacts with its targets by serving as a spectroscopic off-on probe for nitroreductase and a fluorometric competitive substrate for cytochrome P450 . This means that the compound changes its spectroscopic properties upon interaction with these enzymes, allowing for real-time detection of their activity .
Biochemical Pathways
These enzymes are involved in various biochemical pathways, including the reduction of nitro compounds and the metabolism of drugs, respectively .
Pharmacokinetics
Given its role as a substrate for cytochrome p450, it is likely that the compound undergoes metabolic transformations in the body .
Result of Action
The interaction of Methoxyresorufin with nitroreductase and cytochrome P450 results in changes to the compound’s spectroscopic properties . This allows for the real-time detection of these enzymes’ activity, providing valuable information about their role in various biochemical processes .
Action Environment
The action of Methoxyresorufin can be influenced by various environmental factors. For instance, the activity of nitroreductase and cytochrome P450, and thus the efficacy of Methoxyresorufin as a probe or substrate, can be affected by factors such as pH, temperature, and the presence of other compounds .
Biochemical Analysis
Biochemical Properties
Methoxyresorufin is a substrate for the cytochrome P450 (CYP) isoforms CYP1A1 and CYP1A2 . It is O-demethylated by these enzymes, releasing resorufin . This process can be used to quantify CYP1A1/2 activity .
Cellular Effects
Methoxyresorufin plays a significant role in cellular processes, particularly in the determination of cytochrome P4501A activities such as ethoxyresorufin O-deethylase (EROD) and methoxyresorufin O-demethylase (MROD) in liver cells .
Molecular Mechanism
The molecular mechanism of Methoxyresorufin involves its O-demethylation by CYP1A1/2, which releases resorufin . This process allows for the quantification of CYP1A1/2 activity, providing a measure of the enzyme’s function .
Metabolic Pathways
Methoxyresorufin is involved in the metabolic pathways of the cytochrome P450 enzymes CYP1A1 and CYP1A2 . It is O-demethylated by these enzymes, a process that can be used to quantify their activity .
Preparation Methods
Synthetic Routes:
The synthesis of Methoxyresorufin involves the following steps:
O-Demethylation: Methoxyresorufin is synthesized by O-demethylation of , resulting in the removal of the methoxy group (CH₃O) from the resorufin molecule.
Reaction Conditions: The O-demethylation reaction typically occurs under mild conditions using specific enzymes (CYP1A1 or CYP1A2) in biological systems.
Industrial Production:
Methoxyresorufin is not produced industrially on a large scale. Instead, it serves as a valuable research tool in laboratories.
Chemical Reactions Analysis
Methoxyresorufin undergoes enzymatic O-demethylation by CYP1A1/2, leading to the release of Resorufin . The fluorescence of resorufin can be quantified to assess CYP1A1/2 activity . Common reagents and conditions for this reaction include the use of liver microsomes, NADPH (cofactor), and specific enzyme isoforms.
Scientific Research Applications
Chemical Applications
Fluorescent Dye
7-Methoxy-3H-phenoxazin-3-one is extensively utilized as a fluorescent dye in chemical assays and experiments. Its ability to absorb light at specific wavelengths and emit light at different wavelengths makes it suitable for fluorescence microscopy and other imaging techniques. This property is particularly valuable in studying molecular interactions and cellular processes.
Synthesis of Derivatives
The compound serves as a precursor for synthesizing various aminophenoxazinone derivatives, which have been evaluated for their phytotoxicity and potential applications in agriculture . This includes the synthesis of derivatives that exhibit selective activity against certain plant species, showcasing its utility in agrochemical formulations.
Biological Applications
Histone Deacetylase Inhibition
Research indicates that this compound acts as a histone deacetylase (HDAC) inhibitor, which is significant for cancer therapy. HDAC inhibitors play a crucial role in modifying chromatin structure and regulating gene expression, making them potential agents for treating various cancers . In vitro studies have demonstrated that this compound can inhibit HDAC activity in tumor cells while exhibiting low toxicity towards non-malignant cells .
Allelopathic Effects
This compound also exhibits allelopathic properties, influencing the growth of neighboring plants by interfering with their molecular and cellular processes. This characteristic is particularly relevant in understanding plant competition and developing natural herbicides .
Medical Applications
Diagnostic Imaging
In medical research, this compound is explored for its potential use in diagnostic imaging. Its fluorescent properties allow it to serve as a marker in various medical tests, facilitating the visualization of biological samples.
Anti-inflammatory Properties
Studies suggest that phenoxazinones may possess anti-inflammatory effects alongside their anticancer activities. This dual functionality could lead to the development of new therapeutic strategies for treating inflammatory diseases .
Agricultural Applications
Phytotoxicity Studies
Recent evaluations of aminophenoxazinone derivatives have shown promising results regarding their phytotoxicity on agricultural weeds and crops. These studies are crucial for developing eco-friendly herbicides that can manage weed populations without harming desirable crops .
Summary Table of Applications
Case Study 1: HDAC Inhibition
A study investigated the effects of this compound on hepatoma cell lines (HepG2). The results indicated a concentration-dependent inhibition of HDAC activity, leading to increased acetylation of histones associated with tumor suppression . The compound demonstrated significant cytotoxic effects on cancer cells while maintaining low toxicity on primary human hepatocytes.
Case Study 2: Allelopathic Effects
Research focusing on the allelopathic potential of phenoxazinones revealed that this compound could effectively reduce the competitiveness of neighboring plants by altering their growth patterns through biochemical pathways . This finding supports its potential application as a natural herbicide.
Comparison with Similar Compounds
While Methoxyresorufin is unique due to its specific interaction with CYP1A1/2, other related compounds include:
Resorufin: The parent compound from which Methoxyresorufin is derived.
Benzyloxyresorufin: Another substrate preferentially metabolized by CYP2B in rodents.
Biological Activity
7-Methoxy-3H-phenoxazin-3-one, also known as 2-amino-7-methoxy-3H-phenoxazin-3-one, is a compound that has garnered attention in the fields of medicinal chemistry and pharmacology. This article explores its biological activities, focusing on its potential as an antimicrobial and anticancer agent, along with its mechanisms of action and relevant research findings.
This compound belongs to the phenoxazinone class of compounds, characterized by a phenolic structure that can influence various biological activities. Its chemical structure allows it to interact with multiple biological targets, making it a candidate for therapeutic applications.
Anticancer Properties
Research indicates that this compound exhibits significant anticancer properties. It has been studied for its effects on various cancer cell lines:
-
Cytotoxicity : The compound demonstrates cytotoxic effects against several cancer types, including gastric and colon cancers. For instance, studies show that it can induce apoptosis in cancer cells by altering the intracellular pH and disrupting mitochondrial function. The half maximal inhibitory concentration (IC50) values for different cancer cell lines have been reported as follows:
These values indicate potent activity at relatively low concentrations .
Cell Line IC50 (µM) MKN45 (Gastric) 6–12 HT-29 (Colon) 16.7 LoVo-1 (Colon) 20.03
The mechanism by which this compound exerts its anticancer effects involves several pathways:
- pH Modulation : It has been shown to significantly decrease the intracellular pH of cancer cells, which is linked to the induction of apoptotic pathways .
- Histone Deacetylase Inhibition : The compound acts as a histone deacetylase (HDAC) inhibitor, leading to changes in gene expression that promote apoptosis .
- Mitochondrial Dysfunction : Treatment with this compound causes depolarization of mitochondria, activating caspases and triggering apoptotic events .
Antimicrobial Activity
In addition to its anticancer properties, this compound has been investigated for its antimicrobial potential. Studies have shown that it possesses activity against various bacterial strains, suggesting its utility in treating infections .
Case Studies
Several case studies highlight the effectiveness of this compound in preclinical models:
- Gastric Cancer Model : In a study involving human gastric cancer cell lines, treatment with the compound resulted in a significant reduction in cell viability and an increase in apoptosis markers compared to untreated controls .
- Colon Cancer Model : Another study demonstrated that administration of this compound led to reduced tumor growth in mouse models of colon cancer, correlating with improved metabolic profiles and reduced adipose tissue mass .
Properties
IUPAC Name |
7-methoxyphenoxazin-3-one | |
---|---|---|
Source | PubChem | |
URL | https://pubchem.ncbi.nlm.nih.gov | |
Description | Data deposited in or computed by PubChem | |
InChI |
InChI=1S/C13H9NO3/c1-16-9-3-5-11-13(7-9)17-12-6-8(15)2-4-10(12)14-11/h2-7H,1H3 | |
Source | PubChem | |
URL | https://pubchem.ncbi.nlm.nih.gov | |
Description | Data deposited in or computed by PubChem | |
InChI Key |
KNYYMGDYROYBRE-UHFFFAOYSA-N | |
Source | PubChem | |
URL | https://pubchem.ncbi.nlm.nih.gov | |
Description | Data deposited in or computed by PubChem | |
Canonical SMILES |
COC1=CC2=C(C=C1)N=C3C=CC(=O)C=C3O2 | |
Source | PubChem | |
URL | https://pubchem.ncbi.nlm.nih.gov | |
Description | Data deposited in or computed by PubChem | |
Molecular Formula |
C13H9NO3 | |
Source | PubChem | |
URL | https://pubchem.ncbi.nlm.nih.gov | |
Description | Data deposited in or computed by PubChem | |
DSSTOX Substance ID |
DTXSID80205859 | |
Record name | 7-Methoxyresorufin | |
Source | EPA DSSTox | |
URL | https://comptox.epa.gov/dashboard/DTXSID80205859 | |
Description | DSSTox provides a high quality public chemistry resource for supporting improved predictive toxicology. | |
Molecular Weight |
227.21 g/mol | |
Source | PubChem | |
URL | https://pubchem.ncbi.nlm.nih.gov | |
Description | Data deposited in or computed by PubChem | |
CAS No. |
5725-89-3 | |
Record name | 7-Methoxyresorufin | |
Source | ChemIDplus | |
URL | https://pubchem.ncbi.nlm.nih.gov/substance/?source=chemidplus&sourceid=0005725893 | |
Description | ChemIDplus is a free, web search system that provides access to the structure and nomenclature authority files used for the identification of chemical substances cited in National Library of Medicine (NLM) databases, including the TOXNET system. | |
Record name | 7-Methoxyresorufin | |
Source | EPA DSSTox | |
URL | https://comptox.epa.gov/dashboard/DTXSID80205859 | |
Description | DSSTox provides a high quality public chemistry resource for supporting improved predictive toxicology. | |
Synthesis routes and methods
Procedure details
Retrosynthesis Analysis
AI-Powered Synthesis Planning: Our tool employs the Template_relevance Pistachio, Template_relevance Bkms_metabolic, Template_relevance Pistachio_ringbreaker, Template_relevance Reaxys, Template_relevance Reaxys_biocatalysis model, leveraging a vast database of chemical reactions to predict feasible synthetic routes.
One-Step Synthesis Focus: Specifically designed for one-step synthesis, it provides concise and direct routes for your target compounds, streamlining the synthesis process.
Accurate Predictions: Utilizing the extensive PISTACHIO, BKMS_METABOLIC, PISTACHIO_RINGBREAKER, REAXYS, REAXYS_BIOCATALYSIS database, our tool offers high-accuracy predictions, reflecting the latest in chemical research and data.
Strategy Settings
Precursor scoring | Relevance Heuristic |
---|---|
Min. plausibility | 0.01 |
Model | Template_relevance |
Template Set | Pistachio/Bkms_metabolic/Pistachio_ringbreaker/Reaxys/Reaxys_biocatalysis |
Top-N result to add to graph | 6 |
Feasible Synthetic Routes
Disclaimer and Information on In-Vitro Research Products
Please be aware that all articles and product information presented on BenchChem are intended solely for informational purposes. The products available for purchase on BenchChem are specifically designed for in-vitro studies, which are conducted outside of living organisms. In-vitro studies, derived from the Latin term "in glass," involve experiments performed in controlled laboratory settings using cells or tissues. It is important to note that these products are not categorized as medicines or drugs, and they have not received approval from the FDA for the prevention, treatment, or cure of any medical condition, ailment, or disease. We must emphasize that any form of bodily introduction of these products into humans or animals is strictly prohibited by law. It is essential to adhere to these guidelines to ensure compliance with legal and ethical standards in research and experimentation.