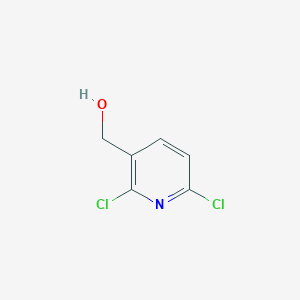
(2,6-Dichloropyridin-3-yl)methanol
Overview
Description
(2,6-Dichloropyridin-3-yl)methanol is a chemical compound with the molecular formula C₆H₅Cl₂NO and a molecular weight of 178.02 g/mol . It is a derivative of pyridine, characterized by the presence of two chlorine atoms at the 2 and 6 positions and a hydroxymethyl group at the 3 position of the pyridine ring. This compound is often used as an intermediate in the synthesis of various pharmaceuticals and agrochemicals.
Preparation Methods
Synthetic Routes and Reaction Conditions
(2,6-Dichloropyridin-3-yl)methanol can be synthesized through several methods. One common method involves the reaction of 2,6-dichloropyridine with formaldehyde in the presence of a base such as sodium hydroxide. The reaction proceeds via nucleophilic addition of the hydroxymethyl group to the pyridine ring, followed by reduction to yield the desired product .
Another method involves the use of Dess-Martin periodane as an oxidizing agent. In this method, this compound is dissolved in dichloromethane, and Dess-Martin periodane is added at room temperature. The reaction mixture is stirred for a specified period, typically around 2 hours, to achieve the desired conversion .
Industrial Production Methods
Industrial production of this compound typically involves large-scale synthesis using similar methods as described above. The choice of method depends on factors such as cost, availability of reagents, and desired purity of the final product. Optimization of reaction conditions, such as temperature, solvent, and reaction time, is crucial to maximize yield and minimize by-products.
Chemical Reactions Analysis
Types of Reactions
(2,6-Dichloropyridin-3-yl)methanol undergoes various chemical reactions, including:
Oxidation: The hydroxymethyl group can be oxidized to a carboxylic acid or aldehyde using oxidizing agents such as potassium permanganate or chromium trioxide.
Reduction: The compound can be reduced to the corresponding amine using reducing agents like lithium aluminum hydride.
Substitution: The chlorine atoms on the pyridine ring can be substituted with other nucleophiles, such as amines or thiols, under appropriate conditions.
Common Reagents and Conditions
Oxidation: Potassium permanganate in an acidic medium or chromium trioxide in acetic acid.
Reduction: Lithium aluminum hydride in anhydrous ether.
Substitution: Nucleophiles such as amines or thiols in the presence of a base like sodium hydroxide or potassium carbonate.
Major Products Formed
Oxidation: (2,6-Dichloropyridin-3-yl)carboxylic acid or (2,6-Dichloropyridin-3-yl)aldehyde.
Reduction: (2,6-Dichloropyridin-3-yl)methanamine.
Substitution: Various substituted pyridines depending on the nucleophile used.
Scientific Research Applications
Chemical Synthesis
(2,6-Dichloropyridin-3-yl)methanol serves as an important intermediate in the synthesis of various organic compounds. Its applications in chemical synthesis include:
- Pharmaceuticals : Utilized in the development of drugs due to its structural similarity to biologically active molecules.
- Agrochemicals : Employed in the synthesis of pesticides and herbicides.
Case Study: Synthesis of Antiviral Agents
Research has demonstrated that derivatives of this compound can inhibit viral replication. For example, studies targeting Hepatitis B virus capsid assembly illustrate its potential as a lead compound for antiviral drug development.
Biological Applications
The compound's biological activity has made it a subject of interest in various research areas:
- Enzyme Inhibition : Investigated for its potential to inhibit specific enzymes involved in disease pathways.
- Receptor Modulation : Its structure allows for interactions with biological receptors, making it relevant in pharmacological studies.
Compound Derivative | Target Disease | Activity Type | Reference |
---|---|---|---|
This compound | Hepatitis B | Viral replication inhibition | |
2-(2,6-Dichloropyridin-3-yl)ethanol | Antifungal | Antimicrobial activity | |
(3-Amino-2,6-dichloropyridin-4-yl)methanol | Inflammatory diseases | Anti-inflammatory |
Medical Applications
In medicine, this compound has been explored for its potential therapeutic effects:
- Drug Development : It acts as a precursor for synthesizing compounds targeting various diseases.
Case Study: Development of Anti-Cancer Agents
Research indicates that modifications to the this compound framework can yield compounds with enhanced efficacy against cancer cells. Studies have shown promising results in preclinical trials.
Industrial Applications
The compound is also utilized in industrial settings:
- Agrochemicals Production : Used in formulating pesticides and herbicides that enhance crop yield.
Data Table: Industrial Uses of this compound
Application Area | Specific Use |
---|---|
Agrochemicals | Synthesis of pesticides |
Material Science | Development of specialty chemicals |
Environmental Monitoring | Tracers for pollution studies |
Environmental Applications
This compound can be employed in environmental studies:
- Pollution Control : Used as markers to trace pollutants and study their environmental impact.
Case Study: Environmental Tracing
Recent studies have utilized this compound as a tracer in water samples to monitor contamination levels and movement of pollutants through ecosystems.
Mechanism of Action
The mechanism of action of (2,6-Dichloropyridin-3-yl)methanol depends on its specific application. In biological systems, it may act as an enzyme inhibitor or receptor modulator by binding to active sites or receptor domains, thereby altering the activity of the target molecule. The presence of chlorine atoms and the hydroxymethyl group can influence its binding affinity and specificity.
Comparison with Similar Compounds
Similar Compounds
(2,6-Dichloropyridine): Lacks the hydroxymethyl group, making it less reactive in certain chemical reactions.
(3-Hydroxymethylpyridine): Lacks the chlorine atoms, resulting in different chemical and biological properties.
(2,6-Dichloro-4-pyridinol): Contains a hydroxyl group at the 4 position instead of the 3 position, leading to different reactivity and applications.
Uniqueness
(2,6-Dichloropyridin-3-yl)methanol is unique due to the presence of both chlorine atoms and the hydroxymethyl group, which confer distinct chemical reactivity and biological activity. This combination allows for versatile applications in various fields, including organic synthesis, medicinal chemistry, and agrochemical production.
Biological Activity
(2,6-Dichloropyridin-3-yl)methanol is a compound of significant interest in medicinal chemistry due to its diverse biological activities. This article explores its mechanisms of action, applications in drug development, and comparative biological activity with related compounds.
Chemical Structure and Properties
This compound features a pyridine ring substituted with two chlorine atoms at the 2 and 6 positions and a hydroxymethyl group at the 3 position. This unique structure contributes to its biological activity by enhancing its binding affinity to various biological targets.
The biological activity of this compound is primarily attributed to its role as an enzyme inhibitor and receptor modulator . The compound can bind to active sites or receptor domains, altering the activity of target molecules. The presence of chlorine atoms and the hydroxymethyl group significantly influence its binding properties and specificity for various targets, including viral proteins and enzymes involved in disease pathways.
Biological Activity
Research indicates that this compound exhibits notable biological activities:
- Antiviral Activity : It has been investigated for its potential to inhibit viral replication, particularly in Hepatitis B virus capsid assembly. This inhibition is facilitated by its structural features that enhance binding to viral proteins.
- Enzyme Inhibition : The compound has shown promise as an inhibitor for various enzymes, making it a candidate for drug development targeting diseases such as cancer and infections.
Comparative Analysis with Related Compounds
To better understand the unique properties of this compound, a comparative analysis with structurally similar compounds is presented in the table below:
Compound Name | Structural Features | Biological Activity |
---|---|---|
(2-Chloropyridin-3-yl)methanol | One chlorine atom on pyridine | Limited antiviral activity |
(2,4-Dichloropyridin-3-yl)methanol | Two chlorine atoms at different positions | Antimicrobial properties |
(3-Pyridyl)methanol | No chlorine substitution | Lower binding affinity |
(2-Methylpyridin-3-yl)methanol | Methyl group instead of chlorine | Different pharmacological profile |
This compound | Two chlorine atoms and hydroxymethyl group | Significant antiviral and enzyme inhibition |
This table illustrates that the combination of two chlorine atoms and a hydroxymethyl group in this compound enhances its biological activity compared to other similar compounds.
Case Studies
- Antiviral Research : A study focused on the compound's effectiveness against Hepatitis B demonstrated that it inhibited viral replication by interfering with capsid assembly. The study highlighted the potential for developing antiviral therapies based on this compound.
- Cancer Research : Another investigation explored its role as an enzyme inhibitor in cancer cell lines. Results indicated that this compound effectively reduced cell proliferation by targeting specific enzymes involved in tumor growth .
Q & A
Q. Basic Synthesis and Optimization
Q. Q1. What are the established synthetic routes for (2,6-dichloropyridin-3-yl)methanol, and how do reaction conditions influence yield?
A1. The compound is synthesized via nucleophilic substitution or oxidation-reduction strategies. For example, this compound derivatives are prepared using LDA (lithium diisopropylamide) and aldehydes, followed by purification via flash chromatography (0–40% i-Hex-EtOAc gradient) . Critical parameters include temperature control (−78°C for LDA reactions), stoichiometric ratios, and solvent choice (THF or DMF). Yields vary significantly (23–80%), emphasizing the need for precise reagent addition and inert atmospheres .
Q. Purification and Characterization
Q. Q2. What chromatographic and spectroscopic methods are recommended for purifying and characterizing this compound?
A2. Flash chromatography on silica gel with gradients (e.g., 0–20% i-Hex-EtOAc) effectively removes byproducts . Characterization requires HPLC-MS (electrospray ionization) for molecular ion detection ([M−H]+ at m/z 280/282) and 1H NMR (δ 2.5–7.5 ppm for pyridine ring protons and hydroxyl groups). IR spectroscopy (e.g., ν~3057 cm⁻¹ for C-H stretching) confirms functional groups .
Q. Stability and Storage
Q. Q3. How should this compound be stored to prevent degradation, and what are its stability-limiting factors?
A3. Store under inert gas (N₂/Ar) at −20°C in amber vials to avoid moisture absorption and photodegradation. Stability is influenced by the electron-withdrawing Cl substituents, which reduce susceptibility to oxidation but may promote hydrolysis under acidic/basic conditions. Monitor purity via HPLC; degradation products include ketones (e.g., (2,6-dichloropyridin-3-yl)methanone) .
Q. Advanced Mechanistic Insights
Q. Q4. How can computational modeling predict the reactivity of this compound in nucleophilic reactions?
A4. DFT calculations (B3LYP/6-31G*) model the electron-deficient pyridine ring, showing enhanced electrophilicity at C4 due to Cl substituents. Solvent effects (e.g., DMSO polarity) stabilize transition states in SNAr reactions. Molecular docking studies further predict binding affinities for biological targets (e.g., β-lactamases) .
Q. Applications in Drug Discovery
Q. Q5. What role does this compound play as a building block in β-lactamase inhibitor design?
A5. It serves as a core scaffold for OXA-48 β-lactamase inhibitors. The dichloropyridine moiety enhances binding to the enzyme’s active site, while the hydroxymethyl group allows functionalization (e.g., silylation with tert-butyldimethylsilyl chloride for improved lipophilicity) . Structure-activity relationship (SAR) studies prioritize substituents at C3 and C5 for potency .
Q. Handling Contradictory Data
Q. Q6. How should researchers address discrepancies in reported synthetic yields or spectroscopic data?
A6. Cross-validate methods: Replicate reactions under identical conditions (e.g., LDA equivalents, reaction time). Use high-resolution mass spectrometry (HRMS) to confirm molecular formulas and 2D NMR (COSY, HSQC) for structural elucidation. Contradictions in yields (e.g., 23% vs. 80%) may arise from purification efficiency or side reactions (e.g., over-oxidation to ketones) .
Q. Derivative Synthesis and Activity
Q. Q7. What strategies optimize the synthesis of this compound derivatives for enhanced bioactivity?
A7. Introduce substituents via:
- Mitsunobu reactions : Convert the hydroxyl group to ethers using DIAD/TPP.
- Oxidation : Dess-Martin periodinane oxidizes the alcohol to ketones for further functionalization .
Derivatives like cyclopentyl(2,6-dichloro-5-methylpyridin-3-yl)methanol show improved pharmacokinetic profiles (logP ~2.59) and thermal stability (predicted bp 402°C) .
Q. Safety and Hazard Mitigation
Q. Q8. What safety protocols are essential when handling this compound?
A8. Use PPE (gloves, goggles) due to potential skin/eye irritation. Avoid inhalation; work in a fume hood. Waste disposal must follow halogenated organic compound guidelines. LC-MS monitoring ensures reaction completion, minimizing exposure to unreacted starting materials (e.g., toxic aldehydes) .
Q. Analytical Challenges
Q. Q9. How can researchers overcome low ionization efficiency in mass spectrometry for this compound?
A9. Employ derivatization: Trimethylsilylation of the hydroxyl group enhances volatility and ionization in GC-MS. Alternatively, use matrix-assisted laser desorption/ionization (MALDI) with α-cyano-4-hydroxycinnamic acid as the matrix .
Q. Comparative Reactivity
Q. Q10. How does the reactivity of this compound differ from its fluoro or methyl-substituted analogs?
A10. Chlorine’s strong electron-withdrawing effect accelerates nucleophilic aromatic substitution compared to methyl groups. Fluorine analogs (e.g., (6-fluoro-2-(trifluoromethyl)pyridin-3-yl)methanol) exhibit higher metabolic stability but lower solubility due to trifluoromethyl hydrophobicity .
Properties
IUPAC Name |
(2,6-dichloropyridin-3-yl)methanol | |
---|---|---|
Source | PubChem | |
URL | https://pubchem.ncbi.nlm.nih.gov | |
Description | Data deposited in or computed by PubChem | |
InChI |
InChI=1S/C6H5Cl2NO/c7-5-2-1-4(3-10)6(8)9-5/h1-2,10H,3H2 | |
Source | PubChem | |
URL | https://pubchem.ncbi.nlm.nih.gov | |
Description | Data deposited in or computed by PubChem | |
InChI Key |
FWEVVZQDRPWAND-UHFFFAOYSA-N | |
Source | PubChem | |
URL | https://pubchem.ncbi.nlm.nih.gov | |
Description | Data deposited in or computed by PubChem | |
Canonical SMILES |
C1=CC(=NC(=C1CO)Cl)Cl | |
Source | PubChem | |
URL | https://pubchem.ncbi.nlm.nih.gov | |
Description | Data deposited in or computed by PubChem | |
Molecular Formula |
C6H5Cl2NO | |
Source | PubChem | |
URL | https://pubchem.ncbi.nlm.nih.gov | |
Description | Data deposited in or computed by PubChem | |
DSSTOX Substance ID |
DTXSID30482830 | |
Record name | (2,6-dichloropyridin-3-yl)methanol | |
Source | EPA DSSTox | |
URL | https://comptox.epa.gov/dashboard/DTXSID30482830 | |
Description | DSSTox provides a high quality public chemistry resource for supporting improved predictive toxicology. | |
Molecular Weight |
178.01 g/mol | |
Source | PubChem | |
URL | https://pubchem.ncbi.nlm.nih.gov | |
Description | Data deposited in or computed by PubChem | |
CAS No. |
55304-90-0 | |
Record name | (2,6-dichloropyridin-3-yl)methanol | |
Source | EPA DSSTox | |
URL | https://comptox.epa.gov/dashboard/DTXSID30482830 | |
Description | DSSTox provides a high quality public chemistry resource for supporting improved predictive toxicology. | |
Synthesis routes and methods I
Procedure details
Synthesis routes and methods II
Procedure details
Synthesis routes and methods III
Procedure details
Synthesis routes and methods IV
Procedure details
Synthesis routes and methods V
Procedure details
Disclaimer and Information on In-Vitro Research Products
Please be aware that all articles and product information presented on BenchChem are intended solely for informational purposes. The products available for purchase on BenchChem are specifically designed for in-vitro studies, which are conducted outside of living organisms. In-vitro studies, derived from the Latin term "in glass," involve experiments performed in controlled laboratory settings using cells or tissues. It is important to note that these products are not categorized as medicines or drugs, and they have not received approval from the FDA for the prevention, treatment, or cure of any medical condition, ailment, or disease. We must emphasize that any form of bodily introduction of these products into humans or animals is strictly prohibited by law. It is essential to adhere to these guidelines to ensure compliance with legal and ethical standards in research and experimentation.