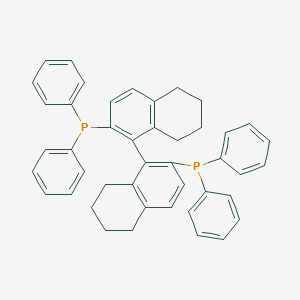
(S)-H8-BINAP
Overview
Description
(S)-H8-BINAP: is a complex organophosphorus compound It is characterized by the presence of two diphenylphosphanyl groups attached to a tetrahydronaphthalene backbone
Preparation Methods
Synthetic Routes and Reaction Conditions: The synthesis of (S)-H8-BINAP typically involves the following steps:
Formation of the Tetrahydronaphthalene Backbone: The initial step involves the preparation of the tetrahydronaphthalene backbone through hydrogenation of naphthalene under high pressure and temperature conditions.
Introduction of Diphenylphosphanyl Groups: The diphenylphosphanyl groups are introduced via a reaction with diphenylphosphine in the presence of a suitable catalyst, such as palladium or platinum complexes. The reaction is typically carried out under an inert atmosphere to prevent oxidation of the phosphine groups.
Industrial Production Methods: Industrial production of this compound may involve continuous flow reactors to ensure consistent reaction conditions and high yields. The use of automated systems for precise control of temperature, pressure, and reactant concentrations is crucial for large-scale synthesis.
Chemical Reactions Analysis
Types of Reactions:
Oxidation: The compound can undergo oxidation reactions, where the phosphine groups are converted to phosphine oxides. Common oxidizing agents include hydrogen peroxide and oxygen.
Reduction: Reduction reactions can convert phosphine oxides back to phosphines using reducing agents such as lithium aluminum hydride.
Substitution: The compound can participate in substitution reactions where one or more hydrogen atoms on the tetrahydronaphthalene backbone are replaced by other functional groups. This can be achieved using halogenating agents like bromine or chlorine.
Common Reagents and Conditions:
Oxidation: Hydrogen peroxide, oxygen, and other peroxides.
Reduction: Lithium aluminum hydride, sodium borohydride.
Substitution: Halogenating agents such as bromine, chlorine, and iodine.
Major Products:
Oxidation: Phosphine oxides.
Reduction: Regenerated phosphines.
Substitution: Halogenated tetrahydronaphthalene derivatives.
Scientific Research Applications
Chemistry:
Catalysis: The compound is used as a ligand in transition metal catalysis, enhancing the reactivity and selectivity of catalytic processes.
Materials Science: It is employed in the synthesis of advanced materials with unique electronic and optical properties.
Biology and Medicine:
Drug Development: The compound’s ability to form stable complexes with metals makes it a candidate for use in drug delivery systems and as a therapeutic agent.
Industry:
Polymer Production: It is used in the production of specialty polymers with enhanced mechanical and thermal properties.
Electronics: The compound is explored for use in the fabrication of electronic components due to its conductive properties.
Mechanism of Action
The compound exerts its effects primarily through its ability to coordinate with metal centers. The diphenylphosphanyl groups act as electron-donating ligands, stabilizing metal complexes and facilitating various catalytic processes. The molecular targets include transition metals such as palladium, platinum, and rhodium, which are commonly used in catalytic cycles.
Comparison with Similar Compounds
Triphenylphosphine: A widely used ligand in catalysis, but lacks the tetrahydronaphthalene backbone.
1,2-Bis(diphenylphosphino)ethane: Another bidentate ligand with a different backbone structure.
Uniqueness:
Backbone Structure: The tetrahydronaphthalene backbone provides unique steric and electronic properties, differentiating it from other phosphine ligands.
Stability: The compound’s stability under various reaction conditions makes it a versatile ligand in catalysis.
Properties
IUPAC Name |
[1-(2-diphenylphosphanyl-5,6,7,8-tetrahydronaphthalen-1-yl)-5,6,7,8-tetrahydronaphthalen-2-yl]-diphenylphosphane | |
---|---|---|
Source | PubChem | |
URL | https://pubchem.ncbi.nlm.nih.gov | |
Description | Data deposited in or computed by PubChem | |
InChI |
InChI=1S/C44H40P2/c1-5-19-35(20-6-1)45(36-21-7-2-8-22-36)41-31-29-33-17-13-15-27-39(33)43(41)44-40-28-16-14-18-34(40)30-32-42(44)46(37-23-9-3-10-24-37)38-25-11-4-12-26-38/h1-12,19-26,29-32H,13-18,27-28H2 | |
Source | PubChem | |
URL | https://pubchem.ncbi.nlm.nih.gov | |
Description | Data deposited in or computed by PubChem | |
InChI Key |
ANSOKCGDSQQISA-UHFFFAOYSA-N | |
Source | PubChem | |
URL | https://pubchem.ncbi.nlm.nih.gov | |
Description | Data deposited in or computed by PubChem | |
Canonical SMILES |
C1CCC2=C(C1)C=CC(=C2C3=C(C=CC4=C3CCCC4)P(C5=CC=CC=C5)C6=CC=CC=C6)P(C7=CC=CC=C7)C8=CC=CC=C8 | |
Source | PubChem | |
URL | https://pubchem.ncbi.nlm.nih.gov | |
Description | Data deposited in or computed by PubChem | |
Molecular Formula |
C44H40P2 | |
Source | PubChem | |
URL | https://pubchem.ncbi.nlm.nih.gov | |
Description | Data deposited in or computed by PubChem | |
Molecular Weight |
630.7 g/mol | |
Source | PubChem | |
URL | https://pubchem.ncbi.nlm.nih.gov | |
Description | Data deposited in or computed by PubChem | |
CAS No. |
61724-04-7, 139139-86-9 | |
Record name | C.I. Acid Brown 21 | |
Source | European Chemicals Agency (ECHA) | |
URL | https://echa.europa.eu/information-on-chemicals | |
Description | The European Chemicals Agency (ECHA) is an agency of the European Union which is the driving force among regulatory authorities in implementing the EU's groundbreaking chemicals legislation for the benefit of human health and the environment as well as for innovation and competitiveness. | |
Explanation | Use of the information, documents and data from the ECHA website is subject to the terms and conditions of this Legal Notice, and subject to other binding limitations provided for under applicable law, the information, documents and data made available on the ECHA website may be reproduced, distributed and/or used, totally or in part, for non-commercial purposes provided that ECHA is acknowledged as the source: "Source: European Chemicals Agency, http://echa.europa.eu/". Such acknowledgement must be included in each copy of the material. ECHA permits and encourages organisations and individuals to create links to the ECHA website under the following cumulative conditions: Links can only be made to webpages that provide a link to the Legal Notice page. | |
Record name | 139139-86-9 | |
Source | European Chemicals Agency (ECHA) | |
URL | https://echa.europa.eu/information-on-chemicals | |
Description | The European Chemicals Agency (ECHA) is an agency of the European Union which is the driving force among regulatory authorities in implementing the EU's groundbreaking chemicals legislation for the benefit of human health and the environment as well as for innovation and competitiveness. | |
Explanation | Use of the information, documents and data from the ECHA website is subject to the terms and conditions of this Legal Notice, and subject to other binding limitations provided for under applicable law, the information, documents and data made available on the ECHA website may be reproduced, distributed and/or used, totally or in part, for non-commercial purposes provided that ECHA is acknowledged as the source: "Source: European Chemicals Agency, http://echa.europa.eu/". Such acknowledgement must be included in each copy of the material. ECHA permits and encourages organisations and individuals to create links to the ECHA website under the following cumulative conditions: Links can only be made to webpages that provide a link to the Legal Notice page. | |
Retrosynthesis Analysis
AI-Powered Synthesis Planning: Our tool employs the Template_relevance Pistachio, Template_relevance Bkms_metabolic, Template_relevance Pistachio_ringbreaker, Template_relevance Reaxys, Template_relevance Reaxys_biocatalysis model, leveraging a vast database of chemical reactions to predict feasible synthetic routes.
One-Step Synthesis Focus: Specifically designed for one-step synthesis, it provides concise and direct routes for your target compounds, streamlining the synthesis process.
Accurate Predictions: Utilizing the extensive PISTACHIO, BKMS_METABOLIC, PISTACHIO_RINGBREAKER, REAXYS, REAXYS_BIOCATALYSIS database, our tool offers high-accuracy predictions, reflecting the latest in chemical research and data.
Strategy Settings
Precursor scoring | Relevance Heuristic |
---|---|
Min. plausibility | 0.01 |
Model | Template_relevance |
Template Set | Pistachio/Bkms_metabolic/Pistachio_ringbreaker/Reaxys/Reaxys_biocatalysis |
Top-N result to add to graph | 6 |
Feasible Synthetic Routes
Disclaimer and Information on In-Vitro Research Products
Please be aware that all articles and product information presented on BenchChem are intended solely for informational purposes. The products available for purchase on BenchChem are specifically designed for in-vitro studies, which are conducted outside of living organisms. In-vitro studies, derived from the Latin term "in glass," involve experiments performed in controlled laboratory settings using cells or tissues. It is important to note that these products are not categorized as medicines or drugs, and they have not received approval from the FDA for the prevention, treatment, or cure of any medical condition, ailment, or disease. We must emphasize that any form of bodily introduction of these products into humans or animals is strictly prohibited by law. It is essential to adhere to these guidelines to ensure compliance with legal and ethical standards in research and experimentation.