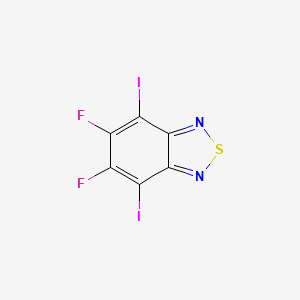
5,6-Difluoro-4,7-diiodo-2,1,3-benzothiadiazole
Overview
Description
5,6-Difluoro-4,7-diiodo-2,1,3-benzothiadiazole is a halogenated heterocyclic aromatic organic compound. It is characterized by the presence of two fluorine atoms and two iodine atoms attached to a benzothiadiazole ring structure. This compound is of interest in various scientific research applications due to its unique chemical properties.
Mechanism of Action
Target of Action
The primary target of 5,6-Difluoro-4,7-diiodo-2,1,3-benzothiadiazole is the electron-rich regions of low-band gap polymer semiconductors . The compound interacts with these targets to influence the electronic properties of the semiconductors .
Mode of Action
This compound acts as an electron-withdrawing unit when embedded into low-band gap polymer semiconductors . The presence of extra fluorine atoms on the benzothiadiazole ring makes the compound more electron-withdrawing . This interaction results in better electron affinity and further lowers the band gap of the semiconducting materials .
Biochemical Pathways
The compound’s interaction with its targets affects the electronic properties of the semiconductors, influencing their performance in devices such as light-emitting diodes and photovoltaic devices . The lowering of the band gap enhances the overall performance of polymer-based bulk-heterojunction (BHJ) solar cells .
Result of Action
The result of the compound’s action is an enhancement in the performance of polymer-based devices. For instance, the introduction of fluorine atoms onto the conjugated polymer backbone has been verified to enhance the overall performance of polymer-based bulk-heterojunction (BHJ) solar cells . This is achieved by lowering the polymer HOMO (Highest Occupied Molecular Orbital) level, resulting in high open-circuit voltages well exceeding 0.7 V .
Biochemical Analysis
Biochemical Properties
5,6-Difluoro-4,7-diiodo-2,1,3-benzothiadiazole plays a crucial role in biochemical reactions due to its ability to interact with a variety of enzymes, proteins, and other biomolecules. This compound is known to interact with enzymes involved in oxidative stress responses, such as superoxide dismutase and catalase. These interactions are primarily mediated through the formation of covalent bonds between the iodine atoms of this compound and the active sites of these enzymes. Additionally, the fluorine atoms in the compound enhance its electron-withdrawing properties, which can influence the reactivity of the enzymes it interacts with .
Cellular Effects
The effects of this compound on various types of cells and cellular processes are profound. This compound has been shown to influence cell signaling pathways, particularly those involved in apoptosis and cell proliferation. By modulating the activity of key signaling proteins such as p53 and Akt, this compound can induce apoptosis in cancer cells while promoting cell survival in normal cells. Furthermore, this compound affects gene expression by altering the transcriptional activity of genes involved in cellular metabolism and stress responses .
Molecular Mechanism
At the molecular level, this compound exerts its effects through several mechanisms. One of the primary mechanisms is the inhibition of enzyme activity by binding to the active sites of target enzymes. This binding is facilitated by the iodine atoms in the compound, which form covalent bonds with the enzyme’s active site residues. Additionally, this compound can modulate gene expression by interacting with transcription factors and altering their binding affinity to DNA. This results in changes in the expression levels of genes involved in various cellular processes .
Temporal Effects in Laboratory Settings
The temporal effects of this compound in laboratory settings have been extensively studied. This compound exhibits high stability under standard laboratory conditions, with minimal degradation over time. Long-term studies have shown that this compound can maintain its biochemical activity for extended periods, making it a reliable tool for in vitro and in vivo experiments. Prolonged exposure to light and high temperatures can lead to gradual degradation of the compound, affecting its efficacy .
Dosage Effects in Animal Models
In animal models, the effects of this compound vary with different dosages. At low doses, this compound has been shown to enhance cellular antioxidant defenses and promote cell survival. At high doses, this compound can induce oxidative stress and cause cellular damage. Threshold effects have been observed, where the compound exhibits beneficial effects up to a certain concentration, beyond which toxic effects become apparent. These findings highlight the importance of optimizing dosage levels for therapeutic applications .
Metabolic Pathways
This compound is involved in several metabolic pathways, primarily those related to oxidative stress and detoxification. This compound interacts with enzymes such as glutathione S-transferase and cytochrome P450, which play key roles in the metabolism and detoxification of xenobiotics. By modulating the activity of these enzymes, this compound can influence metabolic flux and alter the levels of various metabolites in cells .
Transport and Distribution
The transport and distribution of this compound within cells and tissues are mediated by specific transporters and binding proteins. This compound is known to interact with membrane transporters such as ABC transporters, which facilitate its uptake and efflux across cellular membranes. Additionally, binding proteins such as albumin can influence the distribution and localization of this compound within tissues, affecting its bioavailability and efficacy .
Subcellular Localization
The subcellular localization of this compound is critical for its activity and function. This compound is primarily localized in the cytoplasm, where it interacts with various cytoplasmic enzymes and proteins. Additionally, this compound can be targeted to specific subcellular compartments such as the mitochondria and nucleus through post-translational modifications and targeting signals. These localization patterns are essential for the compound’s ability to modulate cellular processes and exert its biochemical effects .
Preparation Methods
Synthetic Routes and Reaction Conditions: The synthesis of 5,6-Difluoro-4,7-diiodo-2,1,3-benzothiadiazole typically involves halogenation reactions of benzothiadiazole derivatives. One common method is the iodination and fluorination of 2,1,3-benzothiadiazole using iodine and fluorinating agents under controlled conditions.
Industrial Production Methods: In an industrial setting, the compound is produced through large-scale halogenation reactions, often involving continuous flow reactors to ensure consistent quality and yield. The process requires careful control of temperature, pressure, and reagent concentrations to achieve high purity and yield.
Types of Reactions:
Oxidation: The compound can undergo oxidation reactions, often resulting in the formation of oxo derivatives.
Reduction: Reduction reactions can lead to the removal of halogen atoms, producing less halogenated derivatives.
Substitution: Substitution reactions are common, where halogen atoms are replaced by other functional groups.
Common Reagents and Conditions:
Oxidation: Common oxidizing agents include potassium permanganate (KMnO4) and hydrogen peroxide (H2O2).
Reduction: Reducing agents like lithium aluminum hydride (LiAlH4) and sodium borohydride (NaBH4) are often used.
Substitution: Various nucleophiles and electrophiles can be used for substitution reactions, depending on the desired product.
Major Products Formed:
Oxidation: Formation of oxo derivatives.
Reduction: Formation of less halogenated derivatives.
Substitution: Formation of various functionalized derivatives.
Scientific Research Applications
Chemistry: In chemistry, 5,6-Difluoro-4,7-diiodo-2,1,3-benzothiadiazole is used as a building block for the synthesis of more complex organic molecules. Its halogenated structure makes it a valuable intermediate in organic synthesis.
Biology: The compound has potential applications in biological research, particularly in the study of halogenated compounds' effects on biological systems. It can be used to investigate the interactions between halogenated molecules and biological targets.
Medicine: In medicinal chemistry, the compound is explored for its potential therapeutic properties. Its unique structure may offer advantages in the design of new drugs, particularly those targeting specific biological pathways.
Industry: In the industry, the compound is used in the development of advanced materials, such as organic semiconductors and photovoltaic devices
Comparison with Similar Compounds
4,7-Dibromo-5,6-difluoro-2,1,3-benzothiadiazole
5,6-Difluoro-2,1,3-benzothiadiazole
4,7-Diiodo-2,1,3-benzothiadiazole
Uniqueness: 5,6-Difluoro-4,7-diiodo-2,1,3-benzothiadiazole is unique due to its combination of fluorine and iodine atoms, which imparts distinct chemical properties compared to its brominated or non-halogenated counterparts. This combination allows for a broader range of chemical reactions and applications.
Properties
IUPAC Name |
5,6-difluoro-4,7-diiodo-2,1,3-benzothiadiazole | |
---|---|---|
Source | PubChem | |
URL | https://pubchem.ncbi.nlm.nih.gov | |
Description | Data deposited in or computed by PubChem | |
InChI |
InChI=1S/C6F2I2N2S/c7-1-2(8)4(10)6-5(3(1)9)11-13-12-6 | |
Source | PubChem | |
URL | https://pubchem.ncbi.nlm.nih.gov | |
Description | Data deposited in or computed by PubChem | |
InChI Key |
WLNFHSOQOLOFQO-UHFFFAOYSA-N | |
Source | PubChem | |
URL | https://pubchem.ncbi.nlm.nih.gov | |
Description | Data deposited in or computed by PubChem | |
Canonical SMILES |
C1(=C(C2=NSN=C2C(=C1F)I)I)F | |
Source | PubChem | |
URL | https://pubchem.ncbi.nlm.nih.gov | |
Description | Data deposited in or computed by PubChem | |
Molecular Formula |
C6F2I2N2S | |
Source | PubChem | |
URL | https://pubchem.ncbi.nlm.nih.gov | |
Description | Data deposited in or computed by PubChem | |
Molecular Weight |
423.95 g/mol | |
Source | PubChem | |
URL | https://pubchem.ncbi.nlm.nih.gov | |
Description | Data deposited in or computed by PubChem | |
Retrosynthesis Analysis
AI-Powered Synthesis Planning: Our tool employs the Template_relevance Pistachio, Template_relevance Bkms_metabolic, Template_relevance Pistachio_ringbreaker, Template_relevance Reaxys, Template_relevance Reaxys_biocatalysis model, leveraging a vast database of chemical reactions to predict feasible synthetic routes.
One-Step Synthesis Focus: Specifically designed for one-step synthesis, it provides concise and direct routes for your target compounds, streamlining the synthesis process.
Accurate Predictions: Utilizing the extensive PISTACHIO, BKMS_METABOLIC, PISTACHIO_RINGBREAKER, REAXYS, REAXYS_BIOCATALYSIS database, our tool offers high-accuracy predictions, reflecting the latest in chemical research and data.
Strategy Settings
Precursor scoring | Relevance Heuristic |
---|---|
Min. plausibility | 0.01 |
Model | Template_relevance |
Template Set | Pistachio/Bkms_metabolic/Pistachio_ringbreaker/Reaxys/Reaxys_biocatalysis |
Top-N result to add to graph | 6 |
Feasible Synthetic Routes
Disclaimer and Information on In-Vitro Research Products
Please be aware that all articles and product information presented on BenchChem are intended solely for informational purposes. The products available for purchase on BenchChem are specifically designed for in-vitro studies, which are conducted outside of living organisms. In-vitro studies, derived from the Latin term "in glass," involve experiments performed in controlled laboratory settings using cells or tissues. It is important to note that these products are not categorized as medicines or drugs, and they have not received approval from the FDA for the prevention, treatment, or cure of any medical condition, ailment, or disease. We must emphasize that any form of bodily introduction of these products into humans or animals is strictly prohibited by law. It is essential to adhere to these guidelines to ensure compliance with legal and ethical standards in research and experimentation.