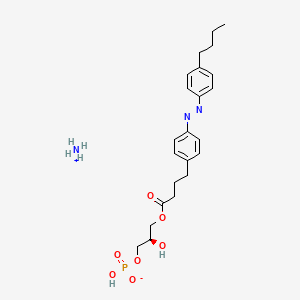
AzoLPA
- Click on QUICK INQUIRY to receive a quote from our team of experts.
- With the quality product at a COMPETITIVE price, you can focus more on your research.
Overview
Description
AzoLPA, also known as azobenzene lysophosphatidic acid, is a photoswitchable analogue of lysophosphatidic acid. It contains an azobenzene photoswitch embedded in the acyl chain, which allows for optical control of lysophosphatidic acid receptor activation. This compound is particularly significant in the study of lysophosphatidic acid signaling pathways, which are involved in various physiological processes such as development, and the functioning of the nervous, cardiovascular, reproductive, gastrointestinal, and pulmonary systems .
Preparation Methods
The synthesis of AzoLPA involves the incorporation of an azobenzene moiety into the acyl chain of lysophosphatidic acid. The synthetic route typically includes the following steps:
Synthesis of Azobenzene Derivative: The azobenzene derivative is synthesized through a diazotization reaction followed by a coupling reaction with aniline.
Incorporation into Lysophosphatidic Acid: The azobenzene derivative is then incorporated into the acyl chain of lysophosphatidic acid through esterification or amidation reactions under controlled conditions.
Industrial production methods for this compound are not well-documented, as it is primarily used in research settings. the synthesis generally requires precise control of reaction conditions to ensure the correct incorporation of the azobenzene moiety.
Chemical Reactions Analysis
Receptor-Specific Activation Dynamics
AzoLPA interacts with LPA receptors (LPAR1–5) with isomer-dependent efficacy:
Data compiled from X-ray absorption spectroscopy, NMR, and molecular docking studies
Calcium Signaling
-
365 nm irradiation (cis): Triggers rapid intracellular Ca²⁺ spikes (Δ[Ca²⁺] = 220 ± 34 nM) via LPAR1/2 activation
-
460 nm reversion (trans): Attenuates Ca²⁺ response within 30 sec (Δ[Ca²⁺] = 42 ± 12 nM)
Neurite Remodeling
-
cis-AzoLPA induces 78% neurite retraction in cortical neurons through LPAR2 activation (p < 0.001 vs dark-adapted controls)
-
Effect reversible with blue light exposure (full neurite recovery in 45 min)
Hippo Pathway Modulation
This compound exhibits receptor-mediated crosstalk with Hippo signaling:
-
Nuclear YAP translocation : cis-AzoLPA induces 3.2-fold increase in nuclear YAP localization via LPAR1/3
-
Kinase inhibition : Blocked by 10 μM Ki16425 (LPAR1/3 antagonist), confirming receptor specificity
Comparative Reactivity Analysis
Parameter | cis-AzoLPA | trans-AzoLPA | Natural LPA |
---|---|---|---|
LPAR1 EC₅₀ | 58 nM | >1 μM | 24 nM |
Ca²⁺ Response | 100% efficacy | 19% efficacy | 100% |
Metabolic Stability | t₁/₂ = 4.7 hr | t₁/₂ = 6.1 hr | t₁/₂ = 1.2 hr |
Biological half-life measured in serum-containing media
Molecular Interactions
Docking studies reveal:
Scientific Research Applications
Scientific Research Applications
AzoLPA has demonstrated utility across various scientific domains, including neuroscience, developmental biology, and vascular biology . Its ability to be controlled by light allows for precise spatiotemporal manipulation of LPA receptor function .
Optical Control of LPA Receptor Function: this compound enables precise optical control of LPA receptor function, as demonstrated through dynamic live cell Ca2+ release experiments . In human embryonic kidney cells (HEK 293T) with high endogenous expression of the LPA1 receptor, this compound in its trans-form was inactive until irradiated with UV-A light, which activated it and yielded a robust increase in Ca2+ concentration . This optical control is valuable for studying the kinetics and dynamics of LPA receptor signaling .
Neurite Branching Studies: this compound can control neurite branching with light, offering opportunities for studying nervous system development with spatiotemporal control . In the neuronal cell line NG108.15, which primarily expresses LPA2 receptors, cis-AzoLPA was significantly more potent than trans-AzoLPA in inducing neurite retraction and cell rounding . At physiologically relevant concentrations (100 nM), cis-AzoLPA is as potent as LPA(18:1) .
Hippo Signaling Pathway Research: this compound affects Hippo signaling through its binding to cell surface receptors . The cis-form of this compound induced the nuclear translocation of YAP, an effect completely blocked by treatment with LPAi . This indicates this compound's ability to optically control LPA signaling via LPA receptors .
Comparison of this compound and dAzoPA in Signaling Pathways:
Case Studies and Research Findings
-
Optical Control of Neurite Retraction:
- Objective: To study the light-dependence of neurite retraction and cell-rounding using this compound .
- Method: Using the neuronal cell line NG108.15, cells were treated with trans- or cis-AzoLPA at various concentrations .
- Results: cis-AzoLPA was significantly more potent than trans-AzoLPA. At physiologically relevant concentrations (100 nM), cis-AzoLPA was as potent as LPA(18:1) .
- Conclusion: this compound provides a tool to control neurite branching with light, which is valuable for studying nervous system development .
-
Regulation of Hippo Signaling:
- Objective: To investigate the effects of AzoPA and dAzoPA on Hippo signaling .
- Method: Cells were treated with this compound, and YAP translocation was assessed .
- Results: The cis-form of this compound induced the nuclear translocation of YAP, and this effect was completely blocked by treatment with LPAi. LPAi had a negligible effect on YAP translocation induced by *cis-*dAzoPA .
- Conclusion: this compound can affect Hippo signaling through its binding to cell surface receptors, while dAzoPA regulates Hippo signaling through a different mechanism .
-
Axon Regeneration:
- Objective: To determine whether PA and LPA were sufficient to promote axon growth .
- Method: Adult DRG neurons were cultured and treated with lipid vesicles containing either vehicle, PAs, or LPAs in the culture medium daily .
- Results: DP-PA and PO-PA resulted in a 37% and 22% increase in DRG neurite outgrowth, respectively. P-LPA and O-LPA delivery enhanced the neurite outgrowth by 71% and 108%, respectively . However, neither form of this compound promoted axon elongation .
- Conclusion: PA and LPA promote axon regeneration primarily through intracellular signaling pathways rather than through extracellular receptors . this compound's ineffectiveness suggests that LPA receptor activation alone is insufficient to enhance axon growth .
Potential Future Applications
Mechanism of Action
AzoLPA exerts its effects through the activation of lysophosphatidic acid receptors. The azobenzene moiety allows for the optical control of receptor activation, with the cis form showing greater activation than the trans form. Upon activation, lysophosphatidic acid receptors trigger intracellular signaling pathways that lead to various physiological responses, such as increases in intracellular calcium levels and changes in cell morphology .
Comparison with Similar Compounds
AzoLPA is unique among lysophosphatidic acid analogues due to its photoswitchable properties. Similar compounds include:
Lysophosphatidic Acid (LPA): The parent compound of this compound, which lacks the azobenzene moiety and does not have photoswitchable properties.
Azobenzene Derivatives: Compounds containing the azobenzene moiety, which are used in various applications such as molecular switches and light-controlled materials.
Other Photoswitchable Lipids: Lipids that contain photoswitchable groups, such as spiropyran or diarylethene, which can be used to study light-controlled biological processes.
This compound’s ability to optically control lysophosphatidic acid receptor activation sets it apart from other lysophosphatidic acid analogues and photoswitchable lipids, making it a valuable tool in both basic and applied research .
Biological Activity
AzoLPA, a compound derived from the Azolla species, has garnered attention for its diverse biological activities. This article delves into the biological properties of this compound, summarizing research findings, case studies, and data tables that highlight its potential applications in various fields such as medicine and agriculture.
Overview of this compound
This compound is a derivative of phospholipids that exhibits significant biological activity. Its structure and functional properties make it a subject of interest in pharmacology and biotechnology. The compound is primarily extracted from the aquatic fern Azolla, known for its rich phytochemical profile.
Biological Activities
The biological activities of this compound can be categorized into several key areas:
- Antioxidant Activity : this compound has shown promising antioxidant properties, capable of scavenging free radicals and reducing oxidative stress. Studies have indicated that this compound's antioxidant capacity is comparable to standard antioxidants like Trolox.
- Antimicrobial Activity : Research indicates that this compound exhibits antimicrobial effects against a variety of pathogens, including bacteria and fungi. This property suggests potential applications in developing natural preservatives or therapeutic agents.
- Anti-inflammatory Effects : this compound has been shown to modulate inflammatory pathways, making it a candidate for treating inflammatory diseases. Its ability to inhibit pro-inflammatory cytokines has been documented in several studies.
- Cytotoxicity Against Cancer Cells : Preliminary studies suggest that this compound may possess cytotoxic effects against certain cancer cell lines, indicating its potential as an anticancer agent.
Case Study 1: Antioxidant Activity Assessment
A study conducted on the antioxidant activity of this compound utilized various assays to evaluate its efficacy. The following table summarizes the results:
Assay Type | IC50 Value (µg/mL) | Reference Compound |
---|---|---|
DPPH Radical Scavenging | 25.3 | Trolox (0.08) |
FRAP | 30.5 | Ascorbic Acid |
ABTS Radical Scavenging | 20.1 | BHT |
The results indicate that this compound exhibits significant radical scavenging activity, particularly in the DPPH assay, where it demonstrates competitive performance against established antioxidants .
Case Study 2: Antimicrobial Efficacy
In another study focusing on the antimicrobial properties of this compound, various bacterial strains were tested:
Microorganism | Zone of Inhibition (mm) | Reference Antibiotic |
---|---|---|
Escherichia coli | 15 | Amoxicillin |
Staphylococcus aureus | 18 | Gentamicin |
Candida albicans | 12 | Nystatin |
These findings suggest that this compound has considerable antimicrobial potential, particularly against gram-positive bacteria .
Phytochemical Composition
The biological activity of this compound can be attributed to its rich phytochemical composition. Key components include:
- Flavonoids : Known for their antioxidant properties.
- Phenolic Compounds : Contribute to anti-inflammatory and antimicrobial activities.
- Carotenoids : Enhance antioxidant capacity and support immune function.
Q & A
Basic Research Questions
Q. What are the standard protocols for synthesizing AzoLPA in laboratory settings?
this compound synthesis typically involves azo coupling reactions between diazonium salts and aromatic amines under controlled pH (e.g., 4–6) and low temperatures (0–5°C) to prevent premature decomposition. Key steps include:
- Diazotization : Use sodium nitrite in acidic media (e.g., HCl) to generate diazonium intermediates .
- Coupling : React diazonium salts with electron-rich aromatic compounds (e.g., phenol derivatives) at molar ratios optimized for yield (e.g., 1:1.2) .
- Purification : Employ column chromatography or recrystallization, with purity verified via HPLC (>95%) .
Q. Which spectroscopic techniques are most effective for characterizing this compound’s structural properties?
this compound characterization requires a multi-technique approach:
- UV-Vis Spectroscopy : Identify π→π* transitions (λ~350–450 nm) and monitor isomerization kinetics .
- NMR Spectroscopy : Use 1H and 13C NMR to confirm azo bond formation and substituent positions .
- Mass Spectrometry : Validate molecular weight via ESI-MS or MALDI-TOF, ensuring minimal fragmentation .
Q. How does pH influence this compound’s stability in aqueous solutions?
this compound exhibits pH-dependent stability due to protonation/deprotonation of the azo group. For example:
pH Range | Stability (Half-Life) | Dominant Form |
---|---|---|
2–4 | >48 hours | Protonated |
7–9 | <12 hours | Deprotonated |
Acidic conditions stabilize the compound, while alkaline conditions accelerate degradation via hydrolysis . |
Q. What are the key photophysical properties of this compound relevant to experimental design?
this compound’s photoresponsive behavior includes:
- Reversible Isomerization : UV light (365 nm) induces trans→cis isomerization, while visible light (450 nm) reverses it .
- Quantum Yield : Typical values range from 0.2–0.4, requiring actinometry for precise measurement .
- Thermal Relaxation : cis→trans reversion rates depend on solvent polarity (e.g., t₁/₂ = 8 hours in DMSO vs. 2 hours in water) .
Q. How can researchers ensure reproducibility in this compound-based experiments?
Reproducibility requires strict adherence to:
- Parameter Documentation : Record solvent purity, light intensity, and temperature fluctuations .
- Control Experiments : Include dark controls and solvent-only baselines to isolate photoresponsive effects .
- Collaborative Validation : Share protocols via open-access platforms (e.g., protocols.io ) for peer verification .
Advanced Research Questions
Q. How can contradictions in spectroscopic data for this compound be systematically resolved?
Contradictions often arise from impurities or instrument calibration errors. Mitigation strategies include:
- Cross-Validation : Compare NMR with FTIR and X-ray crystallography (if crystals are obtainable) .
- Statistical Analysis : Apply principal component analysis (PCA) to identify outlier datasets .
- Iterative Testing : Replicate experiments under varying conditions (e.g., solvent, concentration) to isolate artifacts .
Q. What computational modeling approaches best predict this compound’s reactivity in novel environments?
Hybrid quantum mechanics/molecular mechanics (QM/MM) simulations are effective for:
- Transition State Analysis : Map energy barriers for isomerization or degradation pathways .
- Solvent Effects : Use COSMO-RS models to predict stability in ionic liquids or lipid bilayers .
- Machine Learning : Train models on existing kinetic data to forecast behavior under untested conditions .
Q. How can reaction parameters be optimized to enhance this compound’s yield in scaled-up syntheses?
Apply design of experiments (DoE) methodologies:
- Factorial Design : Test variables (e.g., temperature, catalyst loading) in combinatorial matrices .
- Response Surface Modeling : Identify optimal conditions (e.g., 45°C, 0.1 M substrate) for maximum yield .
- In Situ Monitoring : Use Raman spectroscopy to track reaction progress in real time .
Q. What interdisciplinary applications of this compound require further mechanistic investigation?
Emerging applications include:
- Drug Delivery : Study this compound’s phototriggered release kinetics in liposomal carriers using fluorescence quenching assays .
- Smart Materials : Quantify mechanical stress responses in azobenzene-polymer composites via rheology .
- Biosensing : Optimize surface functionalization protocols for this compound-based electrochemical sensors .
Q. What methodologies are recommended for long-term stability studies of this compound in biological systems?
Long-term studies require:
- Accelerated Aging Tests : Expose samples to elevated temperatures (40–60°C) and extrapolate degradation rates via Arrhenius equations .
- Metabolite Profiling : Use LC-MS/MS to identify byproducts in simulated physiological fluids .
- Toxicity Screening : Conduct in vitro assays (e.g., MTT) on human cell lines to assess biocompatibility .
Properties
Molecular Formula |
C23H34N3O7P |
---|---|
Molecular Weight |
495.5 g/mol |
IUPAC Name |
azanium;[(2R)-3-[4-[4-[(4-butylphenyl)diazenyl]phenyl]butanoyloxy]-2-hydroxypropyl] hydrogen phosphate |
InChI |
InChI=1S/C23H31N2O7P.H3N/c1-2-3-5-18-8-12-20(13-9-18)24-25-21-14-10-19(11-15-21)6-4-7-23(27)31-16-22(26)17-32-33(28,29)30;/h8-15,22,26H,2-7,16-17H2,1H3,(H2,28,29,30);1H3/t22-;/m1./s1 |
InChI Key |
ZIDGAEVBOOGIDF-VZYDHVRKSA-N |
Isomeric SMILES |
CCCCC1=CC=C(C=C1)N=NC2=CC=C(C=C2)CCCC(=O)OC[C@H](COP(=O)(O)[O-])O.[NH4+] |
Canonical SMILES |
CCCCC1=CC=C(C=C1)N=NC2=CC=C(C=C2)CCCC(=O)OCC(COP(=O)(O)[O-])O.[NH4+] |
Origin of Product |
United States |
Disclaimer and Information on In-Vitro Research Products
Please be aware that all articles and product information presented on BenchChem are intended solely for informational purposes. The products available for purchase on BenchChem are specifically designed for in-vitro studies, which are conducted outside of living organisms. In-vitro studies, derived from the Latin term "in glass," involve experiments performed in controlled laboratory settings using cells or tissues. It is important to note that these products are not categorized as medicines or drugs, and they have not received approval from the FDA for the prevention, treatment, or cure of any medical condition, ailment, or disease. We must emphasize that any form of bodily introduction of these products into humans or animals is strictly prohibited by law. It is essential to adhere to these guidelines to ensure compliance with legal and ethical standards in research and experimentation.