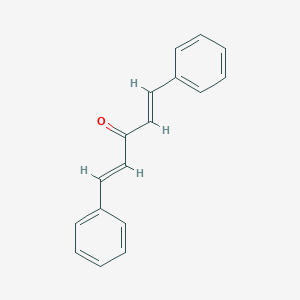
Dibenzylideneacetone
Overview
Description
Dibenzylideneacetone (DBA) is a synthetic α,β-unsaturated ketone featuring two aryl groups conjugated to a central enone system. Its structure (Figure 1) enables diverse applications, including anticancer, antiparasitic, and nonlinear optical (NLO) properties. DBA derivatives induce apoptosis in cervical cancer cells via reactive oxygen species (ROS)-mediated mitochondrial damage, with sub-micromolar EC₅₀ values observed in vitro . Additionally, DBA analogs exhibit potent activity against Trypanosoma brucei, the causative agent of human African trypanosomiasis, with selectivity indices (SI) >10 in HEK293 cytotoxicity screens . Beyond pharmacology, DBA derivatives demonstrate robust NLO responses, such as high first molecular hyperpolarizability (βHRS) and two-photon absorption (TPA), positioning them as candidates for photonic devices .
Preparation Methods
Claisen-Schmidt Condensation: Traditional and Optimized Methods
Sodium Hydroxide-Catalyzed Synthesis
The conventional method, detailed in educational protocols, employs sodium hydroxide (NaOH) as a base catalyst. Benzaldehyde and acetone undergo condensation at 30°C, with stoichiometric ratios critical to minimizing by-products . A typical procedure involves:
-
Combining 10 mL benzaldehyde and 20 mL acetone in a conical flask.
-
Dropwise addition of 2.5 mL NaOH under continuous stirring.
-
Maintaining the reaction at 30°C for 2 hours to ensure complete condensation .
The crude product is acidified with dilute HCl, extracted with chloroform or ether, and crystallized from ethanol. This method yields pale yellow crystals with a melting point of 111–115°C, though reported yields remain low (8.47%) due to challenges in product isolation .
Calcium Hydroxide-Mediated Optimization
Replacing NaOH with calcium hydroxide (Ca(OH)₂) enhances yield and simplifies purification. In a modified approach, diacetone alcohol reacts with benzaldehyde under Ca(OH)₂ catalysis, achieving higher efficiency through improved enolate formation . Key advantages include:
-
Higher yield : Reduced side reactions and easier workup.
-
Cost-effectiveness : Ca(OH)₂ is inexpensive and readily available.
-
Scalability : Suitable for large-scale synthesis without specialized equipment .
This method underscores the role of base strength in optimizing enolate generation, a rate-determining step in aldol condensation.
Aldol Condensation: Parameter Optimization and Mechanistic Insights
Stoichiometric and Temperature Effects
The molar ratio of benzaldehyde to acetone profoundly influences yield. A 2:1 ratio minimizes unreacted benzaldehyde, while excess acetone promotes diketone formation . Temperature control at 30°C balances reaction kinetics and by-product suppression, as higher temperatures accelerate condensation but risk decomposition .
Base Selection and Solvent Systems
Weak bases like sodium acetate (NaOAc) facilitate milder reaction conditions, as evidenced in palladium complex syntheses . In contrast, stronger bases (e.g., NaOH) drive faster enolate formation but necessitate stringent temperature control . Solvent choice also impacts reactivity; ethanol-water mixtures enhance solubility, whereas chloroform aids in product extraction .
Purification and Crystallization Strategies
Post-synthesis purification involves:
-
Liquid-liquid extraction : Chloroform or ether separates this compound from aqueous by-products .
-
Crystallization : Slow cooling in ethanol yields high-purity crystals, with rapid cooling leading to amorphous solids .
-
Vacuum distillation : Removes residual solvents and low-boiling impurities, though thermal sensitivity limits its use .
Optimal purification achieves >95% purity, with melting points consistent across methods (111–115°C) .
Comparative Analysis of Synthetic Approaches
Method | Catalyst | Temperature (°C) | Yield (%) | Purity (MP, °C) |
---|---|---|---|---|
Traditional NaOH | NaOH | 30 | 8.5 | 111–115 |
Ca(OH)₂ Optimization | Ca(OH)₂ | 25–30 | 45–60* | 110–114 |
Palladium Complex Route | NaOAc | 49–53 | N/A | N/A |
*Estimated based on procedural advantages.
The Ca(OH)₂ method outperforms traditional NaOH in yield and scalability, while the palladium-associated route emphasizes ligand stability under inert conditions .
Recent Advances in Reaction Optimization
Design of Experiments (DoE) methodologies identify critical factors such as residence time, reagent equivalents, and mixing efficiency . For instance, increasing benzaldehyde equivalents elevates yield but necessitates precise stoichiometry to avoid poly-condensation . Automated platforms further enable real-time monitoring, reducing optimization timelines from weeks to days .
Chemical Reactions Analysis
Types of Reactions: Dibenzylideneacetone undergoes several types of chemical reactions, including:
Oxidation: It can be oxidized to form benzoic acid and other oxidation products.
Reduction: Reduction of this compound can yield dibenzylacetone.
Cycloaddition: Prolonged exposure to sunlight initiates [2+2] cycloadditions, converting it to a mixture of dimeric and trimeric cyclobutane cycloadducts.
Common Reagents and Conditions:
Oxidation: Common oxidizing agents include potassium permanganate and chromium trioxide.
Reduction: Sodium borohydride or lithium aluminum hydride are typically used as reducing agents.
Cycloaddition: Sunlight or ultraviolet light is used to initiate the reaction.
Major Products:
Oxidation: Benzoic acid and other oxidation products.
Reduction: Dibenzylacetone.
Cycloaddition: Dimeric and trimeric cyclobutane cycloadducts.
Scientific Research Applications
Anticancer Properties
DBA has shown promising results as an anticancer agent through several mechanisms:
- Sensitization to TRAIL-Induced Apoptosis : Research indicates that DBA can sensitize cancer cells to TRAIL (TNF-related apoptosis-inducing ligand), enhancing apoptosis in colon cancer cells. This effect is attributed to the downregulation of anti-apoptotic proteins and the upregulation of death receptors, leading to increased activation of caspases involved in apoptosis .
- Inhibition of Cancer Cell Growth : DBA has been reported to inhibit the growth of various cancer cell lines, including oral and cervical cancer cells. Studies have demonstrated that DBA induces apoptosis via modulation of signaling pathways, such as the inhibition of STAT3 signaling, which is crucial for tumor cell survival .
- Mechanistic Insights : DBA's anticancer effects involve complex interactions at the molecular level. For instance, it has been shown to activate caspases and induce DNA damage, contributing to its efficacy against resistant cancer cell lines .
Case Study: DBA in Cervical Cancer
A recent study highlighted the cytotoxicity of a synthetic DBA variant against cervical cancer cells, demonstrating its potential as a safer alternative to conventional chemotherapeutics. The compound was effective in inducing apoptosis and showed promise for further development as an antitumor agent .
Nonlinear Optical Applications
DBA derivatives have been explored for their nonlinear optical properties, making them suitable for applications in photonics:
- Optical Frequency Conversion : Studies have characterized the nonlinear optical behavior of DBA derivatives, suggesting their potential use in optical frequency conversion devices. The compounds exhibited significant first molecular hyperpolarizability values, indicating their capability to convert infrared light into visible light .
- Potential Photonic Device Development : The findings suggest that DBA derivatives could serve as raw materials for developing advanced photonic devices, particularly those requiring efficient light conversion properties .
Imaging Techniques
DBA has also been investigated for its potential use in imaging applications:
- SPECT Imaging Probes : Research has focused on synthesizing 99mTc-labeled DBA derivatives as potential probes for SPECT (Single Photon Emission Computed Tomography) imaging. These compounds may facilitate the detection of amyloid plaques, which are associated with neurodegenerative diseases .
Summary Table of Applications
Application Area | Description | Key Findings |
---|---|---|
Anticancer Properties | Sensitizes cancer cells to TRAIL-induced apoptosis | Enhanced apoptosis via caspase activation |
Inhibits growth in various cancer types | Effective against resistant cell lines | |
Nonlinear Optics | Potential for optical frequency conversion | High molecular hyperpolarizability |
Imaging Techniques | Development of SPECT imaging probes | Promising results for detecting amyloid plaques |
Mechanism of Action
The mechanism of action of dibenzylideneacetone varies depending on its application:
Comparison with Similar Compounds
Structural Comparison with Related Compounds
DBA shares structural motifs with natural and synthetic bioactive compounds, including curcuminoids, chalcones, and diarylideneacetones. Key differences in stability and activity arise from their backbone modifications (Table 1).
Table 1. Structural and Functional Comparison of DBA with Analogous Compounds
Pharmacological Activity and Selectivity
DBA analogs outperform structurally related compounds in antiparasitic and anticancer activity (Table 2).
Table 2. Pharmacological Activity of DBA vs. Similar Compounds
Key findings:
- DBA’s sub-micromolar EC₅₀ against T.
- In cancer models, DBA derivatives (e.g., A3K2A3) induce apoptosis via ROS-mediated mitochondrial damage, outperforming curcumin’s IC₅₀ by ~10-fold .
Stability and Degradation Pathways
DBA’s α,β-unsaturated ketone linker confers superior stability compared to curcumin’s β-diketone:
- Curcumin : Rapidly degrades via autoxidation to bicyclopentadione in vitro, limiting therapeutic utility .
- DBA : Retains structural integrity under physiological conditions, enabling sustained bioactivity .
Nonlinear Optical Properties
DBA derivatives exhibit strong NLO responses, rivaling benchmark materials (Table 3).
Table 3. NLO Properties of DBA Derivatives vs. Reference Compounds
Key insights:
Biological Activity
Dibenzylideneacetone (DBA) is a compound known for its diverse biological activities, particularly in cancer research and antimicrobial applications. This article explores the mechanisms through which DBA exerts its effects, supported by recent studies and case analyses.
Overview of this compound
This compound is a synthetic compound derived from acetone, characterized by its unique structure that allows for various interactions within biological systems. Its chemical structure can be represented as:
This compound has garnered attention for its potential therapeutic effects, particularly in oncology and infectious diseases.
1. Anticancer Activity
DBA has been extensively studied for its ability to induce apoptosis in cancer cells. The following mechanisms have been identified:
- Sensitization to TRAIL : DBA enhances the sensitivity of cancer cells to TRAIL (TNF-related apoptosis-inducing ligand), promoting apoptosis through the activation of caspases and modulation of pro-apoptotic and anti-apoptotic proteins. Specifically, DBA increases the expression of death receptors DR4 and DR5 while decreasing anti-apoptotic proteins, facilitating TRAIL-induced cell death in colon cancer cells .
- Inhibition of Oncogenic Pathways : DBA has shown efficacy in inhibiting key signaling pathways associated with tumor growth, including:
- Induction of ROS and CHOP : Reactive oxygen species (ROS) play a crucial role in DBA's mechanism by activating CHOP (CCAAT/enhancer-binding protein homologous protein), which further promotes apoptosis .
2. Antimicrobial Activity
DBA exhibits antimicrobial properties against various pathogens. A study demonstrated its effectiveness against several human pathogenic microorganisms:
Microorganism | Zone of Inhibition (mm²) |
---|---|
Candida albicans | 68.23 |
Klebsiella pneumoniae | 49.6 |
Pseudomonas aeruginosa | 37.55 |
Staphylococcus aureus | 0 |
The highest activity was observed against Candida albicans, while Staphylococcus aureus showed resistance . This suggests potential applications for DBA in treating fungal infections.
Case Study 1: DBA in Cervical Cancer
Recent research indicated that DBA derivatives could effectively suppress cervical cancer cell proliferation. The study highlighted the compound's ability to regulate proteins involved in apoptosis without causing significant toxicity to normal cells . This positions DBA as a promising candidate for further development in cervical cancer therapies.
Case Study 2: Antitrypanosomal Activity
A structure-activity relationship study involving DBA analogs revealed potent antitrypanosomal activity against Trypanosoma brucei. The most effective analogs exhibited EC50 values as low as 200 nM, indicating strong potential for treating trypanosomiasis . The presence of a Michael acceptor group was crucial for maintaining this activity.
Q & A
Basic Research Questions
Q. What are the standard synthetic methodologies for preparing dibenzylideneacetone (DBA) in academic laboratories?
DBA is synthesized via the Claisen-Schmidt condensation reaction between benzaldehyde and acetone under alkaline conditions. A typical procedure involves:
- Mixing benzaldehyde (10 mL) and acetone (20 mL) in a conical flask.
- Adding 2.5 mL of sodium hydroxide dropwise under constant stirring in a cold water bath to control exothermicity .
- Filtering the precipitated product and purifying it via recrystallization.
Key Considerations : Excess benzaldehyde ensures high yield. Reaction temperature and base concentration must be optimized to avoid side products like mono-condensed intermediates.
Q. How is DBA utilized as a ligand in palladium-catalyzed cross-coupling reactions?
DBA acts as a stabilizing ligand for palladium(0) in catalysts like Pd₂(dba)₃, widely used in Suzuki, Heck, and Negishi couplings. Its role includes:
- Preventing Pd(0) aggregation, maintaining catalytic activity.
- Enabling ligand exchange during catalysis (e.g., with phosphines).
Experimental Design : Pd₂(dba)₃ is typically prepared by reducing Na₂PdCl₄ with DBA in ethanol, forming a purple-black crystalline solid .
Table 1 : Properties of Pd₂(dba)₃
Property | Value |
---|---|
Molecular Formula | C₅₁H₄₂O₃Pd₂ |
Molecular Weight | 915.72 g/mol |
Melting Point | 152–155°C |
Solubility | Insoluble in water; soluble in THF, DMF |
Q. What spectroscopic techniques are employed to characterize DBA and its metal complexes?
- NMR : ¹H and ¹³C NMR confirm the structure of DBA (e.g., trans-alkene protons at δ 6.5–7.5 ppm) .
- IR : C=O stretching at ~1650 cm⁻¹ and C=C at ~1600 cm⁻¹ .
- UV-Vis : π→π* transitions in DBA derivatives (e.g., λₐᵦₛ ~300 nm) correlate with substituent effects .
Advanced Research Questions
Q. How do structural modifications of DBA derivatives influence their binding affinity to β-amyloid plaques in Alzheimer’s disease research?
Structure-Activity Relationship (SAR) Insights :
- Para-substituents (e.g., iodine, PEG chains) enhance binding to Aβ(1-42) aggregates (Kᵢ = 0.9–7.0 nM) due to steric tolerance.
- Ortho-substituents reduce binding, likely due to steric hindrance .
Methodological Approach : - Radiolabel derivatives (e.g., ¹²⁵I or ¹⁸F) for autoradiography in postmortem AD brain sections.
- Validate selectivity via competitive binding assays with thioflavin T.
Table 2 : Binding Affinity of DBA Derivatives
Derivative (Substituent Position) | Kᵢ (nM) |
---|---|
6 (para-iodo) | 0.9 |
70 (para-PEG) | 4.68 |
Ortho-methyl | >100 |
Q. What mechanistic pathways underlie DBA’s apoptotic effects in cancer cells?
DBA induces apoptosis via:
- ROS-mediated pathways : Upregulation of CHOP, leading to death receptor DR5 activation.
- Sp1 modulation : Downregulation of Sp1 transcription factor, reducing anti-apoptotic proteins (e.g., survivin) .
Experimental Validation : - Treat oral cancer cells with DBA and measure caspase-3/7 activity via fluorometric assays.
- Use siRNA knockdown of Sp1 to confirm its role in apoptosis .
Q. How can researchers address stability challenges when handling Pd₂(dba)₃ in catalytic applications?
Stability Protocols :
- Storage : Under inert gas (N₂/Ar) at –20°C, protected from light and moisture .
- Handling : Use gloveboxes for air-sensitive reactions. Pre-dry solvents (e.g., THF over molecular sieves).
Common Pitfalls : Exposure to air oxidizes Pd(0) to Pd(II), deactivating the catalyst. Confirm activity via control reactions (e.g., biphenyl synthesis in Suzuki coupling) .
Q. Data Contradictions and Resolution
Q. How should discrepancies in reported catalytic activity of Pd₂(dba)₃ across studies be analyzed?
Properties
IUPAC Name |
(1E,4E)-1,5-diphenylpenta-1,4-dien-3-one | |
---|---|---|
Source | PubChem | |
URL | https://pubchem.ncbi.nlm.nih.gov | |
Description | Data deposited in or computed by PubChem | |
InChI |
InChI=1S/C17H14O/c18-17(13-11-15-7-3-1-4-8-15)14-12-16-9-5-2-6-10-16/h1-14H/b13-11+,14-12+ | |
Source | PubChem | |
URL | https://pubchem.ncbi.nlm.nih.gov | |
Description | Data deposited in or computed by PubChem | |
InChI Key |
WMKGGPCROCCUDY-PHEQNACWSA-N | |
Source | PubChem | |
URL | https://pubchem.ncbi.nlm.nih.gov | |
Description | Data deposited in or computed by PubChem | |
Canonical SMILES |
C1=CC=C(C=C1)C=CC(=O)C=CC2=CC=CC=C2 | |
Source | PubChem | |
URL | https://pubchem.ncbi.nlm.nih.gov | |
Description | Data deposited in or computed by PubChem | |
Isomeric SMILES |
C1=CC=C(C=C1)/C=C/C(=O)/C=C/C2=CC=CC=C2 | |
Source | PubChem | |
URL | https://pubchem.ncbi.nlm.nih.gov | |
Description | Data deposited in or computed by PubChem | |
Molecular Formula |
C17H14O | |
Source | PubChem | |
URL | https://pubchem.ncbi.nlm.nih.gov | |
Description | Data deposited in or computed by PubChem | |
DSSTOX Substance ID |
DTXSID0060224, DTXSID401274418 | |
Record name | 1,4-Pentadien-3-one, 1,5-diphenyl- | |
Source | EPA DSSTox | |
URL | https://comptox.epa.gov/dashboard/DTXSID0060224 | |
Description | DSSTox provides a high quality public chemistry resource for supporting improved predictive toxicology. | |
Record name | (1E,4E)-1,5-Diphenyl-1,4-pentadien-3-one | |
Source | EPA DSSTox | |
URL | https://comptox.epa.gov/dashboard/DTXSID401274418 | |
Description | DSSTox provides a high quality public chemistry resource for supporting improved predictive toxicology. | |
Molecular Weight |
234.29 g/mol | |
Source | PubChem | |
URL | https://pubchem.ncbi.nlm.nih.gov | |
Description | Data deposited in or computed by PubChem | |
Physical Description |
Solid; [Merck Index] Yellow crystalline powder; [Acros Organics MSDS] | |
Record name | trans,trans-Dibenzalacetone | |
Source | Haz-Map, Information on Hazardous Chemicals and Occupational Diseases | |
URL | https://haz-map.com/Agents/20558 | |
Description | Haz-Map® is an occupational health database designed for health and safety professionals and for consumers seeking information about the adverse effects of workplace exposures to chemical and biological agents. | |
Explanation | Copyright (c) 2022 Haz-Map(R). All rights reserved. Unless otherwise indicated, all materials from Haz-Map are copyrighted by Haz-Map(R). No part of these materials, either text or image may be used for any purpose other than for personal use. Therefore, reproduction, modification, storage in a retrieval system or retransmission, in any form or by any means, electronic, mechanical or otherwise, for reasons other than personal use, is strictly prohibited without prior written permission. | |
CAS No. |
35225-79-7, 538-58-9 | |
Record name | (1E,4E)-1,5-Diphenyl-1,4-pentadien-3-one | |
Source | CAS Common Chemistry | |
URL | https://commonchemistry.cas.org/detail?cas_rn=35225-79-7 | |
Description | CAS Common Chemistry is an open community resource for accessing chemical information. Nearly 500,000 chemical substances from CAS REGISTRY cover areas of community interest, including common and frequently regulated chemicals, and those relevant to high school and undergraduate chemistry classes. This chemical information, curated by our expert scientists, is provided in alignment with our mission as a division of the American Chemical Society. | |
Explanation | The data from CAS Common Chemistry is provided under a CC-BY-NC 4.0 license, unless otherwise stated. | |
Record name | Dibenzalacetone | |
Source | ChemIDplus | |
URL | https://pubchem.ncbi.nlm.nih.gov/substance/?source=chemidplus&sourceid=0035225797 | |
Description | ChemIDplus is a free, web search system that provides access to the structure and nomenclature authority files used for the identification of chemical substances cited in National Library of Medicine (NLM) databases, including the TOXNET system. | |
Record name | 1,4-Pentadien-3-one, 1,5-diphenyl- | |
Source | EPA Chemicals under the TSCA | |
URL | https://www.epa.gov/chemicals-under-tsca | |
Description | EPA Chemicals under the Toxic Substances Control Act (TSCA) collection contains information on chemicals and their regulations under TSCA, including non-confidential content from the TSCA Chemical Substance Inventory and Chemical Data Reporting. | |
Record name | 1,4-Pentadien-3-one, 1,5-diphenyl- | |
Source | EPA DSSTox | |
URL | https://comptox.epa.gov/dashboard/DTXSID0060224 | |
Description | DSSTox provides a high quality public chemistry resource for supporting improved predictive toxicology. | |
Record name | (1E,4E)-1,5-Diphenyl-1,4-pentadien-3-one | |
Source | EPA DSSTox | |
URL | https://comptox.epa.gov/dashboard/DTXSID401274418 | |
Description | DSSTox provides a high quality public chemistry resource for supporting improved predictive toxicology. | |
Record name | 1,5-diphenylpenta-1,4-dien-3-one | |
Source | European Chemicals Agency (ECHA) | |
URL | https://echa.europa.eu/substance-information/-/substanceinfo/100.007.908 | |
Description | The European Chemicals Agency (ECHA) is an agency of the European Union which is the driving force among regulatory authorities in implementing the EU's groundbreaking chemicals legislation for the benefit of human health and the environment as well as for innovation and competitiveness. | |
Explanation | Use of the information, documents and data from the ECHA website is subject to the terms and conditions of this Legal Notice, and subject to other binding limitations provided for under applicable law, the information, documents and data made available on the ECHA website may be reproduced, distributed and/or used, totally or in part, for non-commercial purposes provided that ECHA is acknowledged as the source: "Source: European Chemicals Agency, http://echa.europa.eu/". Such acknowledgement must be included in each copy of the material. ECHA permits and encourages organisations and individuals to create links to the ECHA website under the following cumulative conditions: Links can only be made to webpages that provide a link to the Legal Notice page. | |
Record name | 1,4-Pentadien-3-one, 1,5-diphenyl-, (1E,4E) | |
Source | European Chemicals Agency (ECHA) | |
URL | https://echa.europa.eu/substance-information/-/substanceinfo/100.126.050 | |
Description | The European Chemicals Agency (ECHA) is an agency of the European Union which is the driving force among regulatory authorities in implementing the EU's groundbreaking chemicals legislation for the benefit of human health and the environment as well as for innovation and competitiveness. | |
Explanation | Use of the information, documents and data from the ECHA website is subject to the terms and conditions of this Legal Notice, and subject to other binding limitations provided for under applicable law, the information, documents and data made available on the ECHA website may be reproduced, distributed and/or used, totally or in part, for non-commercial purposes provided that ECHA is acknowledged as the source: "Source: European Chemicals Agency, http://echa.europa.eu/". Such acknowledgement must be included in each copy of the material. ECHA permits and encourages organisations and individuals to create links to the ECHA website under the following cumulative conditions: Links can only be made to webpages that provide a link to the Legal Notice page. | |
Record name | DIBENZALACETONE | |
Source | FDA Global Substance Registration System (GSRS) | |
URL | https://gsrs.ncats.nih.gov/ginas/app/beta/substances/9QXO7BCY9L | |
Description | The FDA Global Substance Registration System (GSRS) enables the efficient and accurate exchange of information on what substances are in regulated products. Instead of relying on names, which vary across regulatory domains, countries, and regions, the GSRS knowledge base makes it possible for substances to be defined by standardized, scientific descriptions. | |
Explanation | Unless otherwise noted, the contents of the FDA website (www.fda.gov), both text and graphics, are not copyrighted. They are in the public domain and may be republished, reprinted and otherwise used freely by anyone without the need to obtain permission from FDA. Credit to the U.S. Food and Drug Administration as the source is appreciated but not required. | |
Synthesis routes and methods I
Procedure details
Synthesis routes and methods II
Procedure details
Synthesis routes and methods III
Procedure details
Retrosynthesis Analysis
AI-Powered Synthesis Planning: Our tool employs the Template_relevance Pistachio, Template_relevance Bkms_metabolic, Template_relevance Pistachio_ringbreaker, Template_relevance Reaxys, Template_relevance Reaxys_biocatalysis model, leveraging a vast database of chemical reactions to predict feasible synthetic routes.
One-Step Synthesis Focus: Specifically designed for one-step synthesis, it provides concise and direct routes for your target compounds, streamlining the synthesis process.
Accurate Predictions: Utilizing the extensive PISTACHIO, BKMS_METABOLIC, PISTACHIO_RINGBREAKER, REAXYS, REAXYS_BIOCATALYSIS database, our tool offers high-accuracy predictions, reflecting the latest in chemical research and data.
Strategy Settings
Precursor scoring | Relevance Heuristic |
---|---|
Min. plausibility | 0.01 |
Model | Template_relevance |
Template Set | Pistachio/Bkms_metabolic/Pistachio_ringbreaker/Reaxys/Reaxys_biocatalysis |
Top-N result to add to graph | 6 |
Feasible Synthetic Routes
Disclaimer and Information on In-Vitro Research Products
Please be aware that all articles and product information presented on BenchChem are intended solely for informational purposes. The products available for purchase on BenchChem are specifically designed for in-vitro studies, which are conducted outside of living organisms. In-vitro studies, derived from the Latin term "in glass," involve experiments performed in controlled laboratory settings using cells or tissues. It is important to note that these products are not categorized as medicines or drugs, and they have not received approval from the FDA for the prevention, treatment, or cure of any medical condition, ailment, or disease. We must emphasize that any form of bodily introduction of these products into humans or animals is strictly prohibited by law. It is essential to adhere to these guidelines to ensure compliance with legal and ethical standards in research and experimentation.