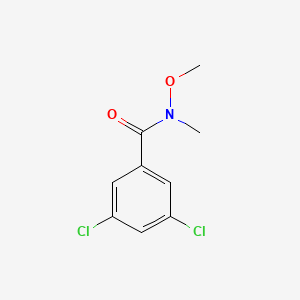
3,5-Dichloro-N-methoxy-N-methylbenzamide
Overview
Description
3,5-Dichloro-N-methoxy-N-methylbenzamide (CAS: 259796-12-8) is a benzamide derivative featuring a dichlorinated aromatic ring and N-methoxy-N-methyl substituents.
Biochemical Analysis
Biochemical Properties
3,5-Dichloro-N-methoxy-N-methylbenzamide plays a significant role in biochemical reactions. It interacts with various enzymes, proteins, and other biomolecules. The compound is known to inhibit certain enzymes, which can affect the biochemical pathways in which these enzymes are involved. For instance, it has been observed to interact with phosphodiesterases, which are enzymes that play a crucial role in cellular signaling pathways . The nature of these interactions often involves binding to the active site of the enzyme, thereby inhibiting its activity.
Cellular Effects
The effects of this compound on various types of cells and cellular processes are profound. It influences cell function by affecting cell signaling pathways, gene expression, and cellular metabolism. For example, the compound has been shown to alter the expression of certain genes involved in metabolic processes . Additionally, it can impact cellular signaling pathways by inhibiting key enzymes, leading to changes in cellular responses and functions.
Molecular Mechanism
At the molecular level, this compound exerts its effects through specific binding interactions with biomolecules. The compound binds to the active sites of enzymes, leading to their inhibition. This inhibition can result in the alteration of biochemical pathways and changes in gene expression. For instance, the binding of this compound to phosphodiesterases inhibits their activity, which in turn affects the levels of cyclic nucleotides in the cell . These changes can have downstream effects on various cellular processes.
Temporal Effects in Laboratory Settings
In laboratory settings, the effects of this compound can change over time. The compound’s stability and degradation are important factors to consider. It has been observed that this compound is relatively stable under certain conditions, but it can degrade over time, leading to changes in its effectiveness . Long-term studies have shown that the compound can have lasting effects on cellular function, even after its degradation products are formed.
Dosage Effects in Animal Models
The effects of this compound vary with different dosages in animal models. At lower doses, the compound may have minimal effects, while higher doses can lead to significant changes in cellular and physiological functions. Studies have shown that there are threshold effects, where a certain dosage is required to observe any noticeable impact . Additionally, at high doses, this compound can exhibit toxic or adverse effects, which are important considerations for its use in research and therapeutic applications.
Metabolic Pathways
This compound is involved in various metabolic pathways. It interacts with enzymes and cofactors that play a role in its metabolism. The compound can affect metabolic flux and alter the levels of metabolites in the cell . Understanding these metabolic pathways is crucial for determining the compound’s overall impact on cellular function and its potential therapeutic applications.
Transport and Distribution
The transport and distribution of this compound within cells and tissues are essential aspects of its biochemical analysis. The compound is transported through specific transporters and binding proteins that facilitate its movement across cellular membranes . Once inside the cell, this compound can accumulate in certain compartments, affecting its localization and activity.
Subcellular Localization
The subcellular localization of this compound is critical for understanding its activity and function. The compound is directed to specific compartments or organelles within the cell through targeting signals or post-translational modifications . This localization can influence the compound’s effectiveness and its interactions with other biomolecules.
Biological Activity
3,5-Dichloro-N-methoxy-N-methylbenzamide is a compound of significant interest in medicinal chemistry due to its diverse biological activities. This article explores its pharmacological properties, mechanisms of action, and potential therapeutic applications based on recent research findings.
- Chemical Formula : C₉H₉Cl₂NO₂
- Molecular Weight : 220.08 g/mol
- Appearance : White to beige powder
- Solubility : Soluble in organic solvents, limited solubility in water
The biological activity of this compound is primarily attributed to its structural similarity to other benzamide derivatives. Its mechanism of action may involve the following:
- Phosphodiesterase Inhibition : Similar compounds have been shown to inhibit phosphodiesterase (PDE) enzymes, particularly PDE10A, which is prevalent in brain tissues. This inhibition can lead to increased levels of cyclic nucleotides, influencing various signaling pathways.
- Hydrogen Bonding Interactions : The methoxy and methyl groups may facilitate hydrogen bonding with biological targets, enhancing the compound's binding affinity and specificity.
Biological Activities
Research indicates that this compound exhibits several biological activities:
- Antimicrobial Activity : Preliminary studies suggest that this compound has antimicrobial properties against various pathogens. It has demonstrated effectiveness against multidrug-resistant strains of bacteria such as Staphylococcus aureus and Mycobacterium species .
- Anticancer Potential : In vitro studies have shown that this compound can inhibit cell proliferation in cancer cell lines, particularly in triple-negative breast cancer (TNBC) models. The compound's IC50 values indicate potent activity against these cancer cells while sparing normal cells .
Case Studies and Research Findings
- Antimicrobial Efficacy :
- Anticancer Activity :
- Pharmacokinetics :
Comparative Analysis with Similar Compounds
Compound Name | Antimicrobial Activity | Anticancer Activity | Mechanism of Action |
---|---|---|---|
This compound | MIC: 4-8 μg/mL | IC50: 0.126 μM | PDE inhibition; hydrogen bonding |
N-Methoxy-N-methylbenzamide | Moderate | Low | Similar PDE inhibition |
2,4-Dichloro-N-methylbenzamide | Low | Moderate | Varies with substitution |
Scientific Research Applications
Chemistry
In organic synthesis, 3,5-Dichloro-N-methoxy-N-methylbenzamide serves as an important intermediate for the development of more complex organic molecules. It facilitates various reactions including nucleophilic substitutions and can be modified to produce derivatives with enhanced properties .
Research has indicated that this compound exhibits potential antimicrobial and anticancer properties. Studies have focused on its interaction with biological targets, particularly its ability to inhibit specific enzymes or receptors involved in disease processes:
- Pharmacological Studies : The compound has been investigated for its effects on phosphodiesterase (PDE) enzymes, which play a crucial role in cellular signaling pathways.
- Case Study : A study demonstrated that derivatives of similar benzamide compounds showed selective binding affinities to opioid receptors, suggesting that modifications to the benzamide structure could enhance therapeutic efficacy against pain .
Pharmaceutical Development
This compound is being explored as a building block in drug development. Its structural characteristics allow it to be tailored for various therapeutic applications:
- Drug Design : It is utilized in the synthesis of novel drugs targeting specific diseases such as cancer and bacterial infections .
- Case Study : In one instance, researchers synthesized a series of benzamide derivatives to evaluate their activity against drug-resistant bacterial strains, with promising results indicating enhanced antimicrobial potency compared to existing treatments .
Industrial Applications
In addition to its research applications, this compound is also employed in the production of specialty chemicals and materials within industrial settings. Its unique reactivity allows for the creation of tailored chemical products that meet specific industry needs.
Q & A
Basic Research Questions
Q. What are the key considerations for synthesizing 3,5-Dichloro-N-methoxy-N-methylbenzamide in a laboratory setting?
- Methodological Answer : Synthesis requires careful selection of reagents and reaction conditions. For example, benzamide derivatives are often synthesized via coupling reactions using acyl chlorides (e.g., 3,5-dichlorobenzoyl chloride) with N-methoxy-N-methylamine in the presence of a base like triethylamine or sodium carbonate . Hazard assessments must precede synthesis, including evaluating risks associated with volatile solvents (e.g., dichloromethane) and mutagenic intermediates, as highlighted in Ames testing protocols . Proper ventilation and personal protective equipment (PPE) are critical due to potential mutagenicity, comparable to benzyl chloride .
Q. How can researchers ensure the purity of this compound post-synthesis?
- Methodological Answer : Purification typically involves column chromatography using gradients of hexanes/ethyl acetate (e.g., 5:1 ratio) to isolate the product . Recrystallization from solvents like acetonitrile or diethyl ether may further enhance purity. Analytical techniques such as <sup>13</sup>C NMR (e.g., δ 168.28 ppm for carbonyl groups) and high-resolution mass spectrometry (HRMS) validate structural integrity . Purity should also be confirmed via HPLC with UV detection at 254 nm.
Q. What spectroscopic methods are most reliable for characterizing this compound?
- Methodological Answer : Nuclear Magnetic Resonance (NMR) spectroscopy is essential. For example, the methoxy group (-OCH3) typically appears as a singlet near δ 3.3–3.5 ppm in <sup>1</sup>H NMR, while aromatic protons in the dichlorophenyl ring resonate as doublets in the δ 7.0–8.0 ppm range . Infrared (IR) spectroscopy can confirm the presence of amide C=O stretches (~1650–1680 cm<sup>-1</sup>) . X-ray crystallography, as demonstrated for structurally similar benzamides, provides definitive confirmation of molecular geometry .
Advanced Research Questions
Q. What strategies are effective in optimizing the yield of this compound under varying reaction conditions?
- Methodological Answer : Yield optimization involves systematic parameter variation:
- Temperature : Reactions are often conducted at 0–25°C to minimize side reactions (e.g., hydrolysis of acyl chlorides) .
- Solvent : Polar aprotic solvents like dichloromethane or acetonitrile improve reagent solubility and reaction kinetics .
- Stoichiometry : A 10–20% excess of N-methoxy-N-methylamine ensures complete conversion of the acyl chloride .
Kinetic studies using in-situ IR or reaction calorimetry can identify rate-limiting steps and guide scale-up protocols .
Q. How should researchers address conflicting spectroscopic data when characterizing novel derivatives of this compound?
- Methodological Answer : Conflicting data require cross-validation:
- Multi-technique analysis : Combine NMR, IR, and X-ray crystallography to resolve ambiguities. For example, crystallographic data for 2-chloro-N-(3,5-dimethylphenyl)benzamide confirmed bond angles and substituent positions that NMR alone could not .
- Computational validation : Density Functional Theory (DFT) calculations predict NMR chemical shifts and vibrational frequencies, which can be compared to experimental data .
- Isotopic labeling : Deuterated analogs or <sup>15</sup>N labeling can clarify complex splitting patterns in NMR spectra .
Q. How do substituent variations on the benzamide core influence biological or physicochemical activity?
- Methodological Answer : Substituent effects are studied via structure-activity relationship (SAR) models:
- Electron-withdrawing groups (e.g., -Cl, -CF3) enhance electrophilicity, potentially increasing reactivity in enzyme inhibition assays .
- Methoxy groups (-OCH3) improve solubility and bioavailability, as seen in analogs like 4-chloro-N-(pyridinylmethyl)benzamide .
- Hydrophobic substituents (e.g., aryl rings) can enhance binding to hydrophobic enzyme pockets, as demonstrated in antifungal benzamide derivatives .
Computational tools like molecular docking (AutoDock) or MD simulations predict binding affinities prior to synthesis .
Comparison with Similar Compounds
Structural and Functional Group Analysis
The table below summarizes key structural differences and similarities between 3,5-Dichloro-N-methoxy-N-methylbenzamide and related benzamide derivatives:
Key Observations:
Substituent Effects :
- Chlorine atoms in this compound enhance electrophilicity compared to methoxy or methyl groups in analogs like 3,5-Dimethoxy-N,N-dimethylbenzamide .
- The N-methoxy-N-methyl group introduces steric hindrance and modulates solubility, contrasting with Propyzamide’s bulky dimethylpropynyl group, which is critical for its herbicidal activity .
- Hydroxy-containing analogs (e.g., N-(2-Hydroxy-1,1-dimethylethyl)-3-methylbenzamide) exhibit chelating capabilities for metal catalysis, a feature absent in the target compound .
- 3,5-Dimethoxy-N,N-dimethylbenzamide’s NMR data (δ 6.48–6.53 ppm for aromatic protons) reflects electron-donating methoxy groups, contrasting with the deshielded aromatic signals expected in dichloro derivatives .
Preparation Methods
General Approach to Weinreb Amides Synthesis
Weinreb amides, including 3,5-dichloro-N-methoxy-N-methylbenzamide, are typically synthesized by coupling carboxylic acids or their activated derivatives with N,O-dimethylhydroxylamine. The key challenge is the activation of the carboxylic acid to facilitate amide bond formation without overreaction or side products.
Preparation from 3,5-Dichlorobenzoic Acid Using Acid Activators
A common and efficient method involves the direct conversion of 3,5-dichlorobenzoic acid into the corresponding Weinreb amide by activating the acid with reagents such as phosphorus oxychloride (POCl3) or methanesulfonyl chloride in the presence of N,O-dimethylhydroxylamine hydrochloride.
-
- 3,5-Dichlorobenzoic acid is treated with POCl3 or methanesulfonyl chloride under controlled temperature conditions.
- N,O-dimethylhydroxylamine hydrochloride is added to the reaction mixture.
- The reaction proceeds via formation of an acid chloride intermediate, which then reacts with the hydroxylamine derivative to form the Weinreb amide.
- Typical reaction temperatures range from 0°C to room temperature.
- The product is isolated by standard extraction and purification techniques.
-
- One-pot synthesis.
- High yields (up to 88% reported in related systems).
- Mild reaction conditions.
- Avoids multi-step procedures and tedious workups.
Alternative Activation via Phosphonium Salt Intermediates
Recent research has introduced the use of in situ generated phosphonium salts for amidation reactions, which can be adapted for Weinreb amide synthesis:
-
- N-chlorophthalimide and triphenylphosphine (PPh3) are combined with the 3,5-dichlorobenzoic acid and N,O-dimethylhydroxylamine.
- This generates reactive phosphonium intermediates that activate the carboxylic acid toward nucleophilic attack by the hydroxylamine.
- The mechanism involves formation of chloro- and imido-phosphonium species as characterized by ^31P NMR and HR-MS.
- This method allows amidation under mild conditions without isolation of acid chlorides.
-
- Avoids harsh reagents like POCl3.
- Provides mechanistic insight for optimization.
- Potential for better selectivity and functional group tolerance.
Note: While this method is promising, specific application to 3,5-dichlorobenzoic acid derivatives requires experimental validation.
Chlorination of N-Methylbenzamide Precursors
A related synthetic route involves:
- Preparation of 2-amino-3,5-dichloro-N-methylbenzamide via a one-pot reaction starting from isatoic anhydride and methylamine.
- Subsequent chlorination using trichloroisocyanuric acid under controlled pH and temperature conditions to introduce chlorine atoms at the 3,5-positions.
- Although this method is described for N-methylbenzamide derivatives, it provides a conceptual framework for preparing chlorinated benzamide intermediates that can be converted to Weinreb amides by further functionalization.
Summary Table of Preparation Methods
Method | Starting Material | Activator/Reagent | Conditions | Yield (%) | Notes |
---|---|---|---|---|---|
POCl3 Activation | 3,5-Dichlorobenzoic acid | POCl3 + N,O-dimethylhydroxylamine HCl | 0°C to RT | Up to 88 | One-pot, mild, high yield |
Methanesulfonyl Chloride Activation | 3,5-Dichlorobenzoic acid | Methanesulfonyl chloride + triethylamine + N,O-dimethylhydroxylamine | RT | ~85 | Efficient for bulky acids |
Phosphonium Salt Activation | 3,5-Dichlorobenzoic acid | N-chlorophthalimide + triphenylphosphine | Mild, in situ generation | Not specified | Mechanistic insight, mild conditions |
Chlorination of N-methylbenzamide | Isatoic anhydride + methylamine | Trichloroisocyanuric acid | 30-50°C, pH 8-13 | High | For chlorinated N-methylbenzamide, precursor |
Research Findings and Considerations
- The use of POCl3 and methanesulfonyl chloride remains the most practical and widely adopted approach for preparing Weinreb amides, including halogenated derivatives like this compound.
- Phosphonium salt methodologies offer innovative alternatives that may improve reaction selectivity and reduce environmental impact but require further development for this specific substrate.
- Chlorination steps using trichloroisocyanuric acid are effective for introducing chlorine substituents on benzamide frameworks, which can then be converted to Weinreb amides through standard amidation protocols.
- Reaction parameters such as temperature, pH, and solvent choice critically influence yield and purity.
- Purification typically involves aqueous workup, pH adjustment, and recrystallization or chromatographic techniques.
Properties
IUPAC Name |
3,5-dichloro-N-methoxy-N-methylbenzamide | |
---|---|---|
Source | PubChem | |
URL | https://pubchem.ncbi.nlm.nih.gov | |
Description | Data deposited in or computed by PubChem | |
InChI |
InChI=1S/C9H9Cl2NO2/c1-12(14-2)9(13)6-3-7(10)5-8(11)4-6/h3-5H,1-2H3 | |
Source | PubChem | |
URL | https://pubchem.ncbi.nlm.nih.gov | |
Description | Data deposited in or computed by PubChem | |
InChI Key |
RDWTUJWXGUBIQW-UHFFFAOYSA-N | |
Source | PubChem | |
URL | https://pubchem.ncbi.nlm.nih.gov | |
Description | Data deposited in or computed by PubChem | |
Canonical SMILES |
CN(C(=O)C1=CC(=CC(=C1)Cl)Cl)OC | |
Source | PubChem | |
URL | https://pubchem.ncbi.nlm.nih.gov | |
Description | Data deposited in or computed by PubChem | |
Molecular Formula |
C9H9Cl2NO2 | |
Source | PubChem | |
URL | https://pubchem.ncbi.nlm.nih.gov | |
Description | Data deposited in or computed by PubChem | |
DSSTOX Substance ID |
DTXSID30730222 | |
Record name | 3,5-Dichloro-N-methoxy-N-methylbenzamide | |
Source | EPA DSSTox | |
URL | https://comptox.epa.gov/dashboard/DTXSID30730222 | |
Description | DSSTox provides a high quality public chemistry resource for supporting improved predictive toxicology. | |
Molecular Weight |
234.08 g/mol | |
Source | PubChem | |
URL | https://pubchem.ncbi.nlm.nih.gov | |
Description | Data deposited in or computed by PubChem | |
CAS No. |
259796-12-8 | |
Record name | 3,5-Dichloro-N-methoxy-N-methylbenzamide | |
Source | EPA DSSTox | |
URL | https://comptox.epa.gov/dashboard/DTXSID30730222 | |
Description | DSSTox provides a high quality public chemistry resource for supporting improved predictive toxicology. | |
Synthesis routes and methods
Procedure details
Retrosynthesis Analysis
AI-Powered Synthesis Planning: Our tool employs the Template_relevance Pistachio, Template_relevance Bkms_metabolic, Template_relevance Pistachio_ringbreaker, Template_relevance Reaxys, Template_relevance Reaxys_biocatalysis model, leveraging a vast database of chemical reactions to predict feasible synthetic routes.
One-Step Synthesis Focus: Specifically designed for one-step synthesis, it provides concise and direct routes for your target compounds, streamlining the synthesis process.
Accurate Predictions: Utilizing the extensive PISTACHIO, BKMS_METABOLIC, PISTACHIO_RINGBREAKER, REAXYS, REAXYS_BIOCATALYSIS database, our tool offers high-accuracy predictions, reflecting the latest in chemical research and data.
Strategy Settings
Precursor scoring | Relevance Heuristic |
---|---|
Min. plausibility | 0.01 |
Model | Template_relevance |
Template Set | Pistachio/Bkms_metabolic/Pistachio_ringbreaker/Reaxys/Reaxys_biocatalysis |
Top-N result to add to graph | 6 |
Feasible Synthetic Routes
Disclaimer and Information on In-Vitro Research Products
Please be aware that all articles and product information presented on BenchChem are intended solely for informational purposes. The products available for purchase on BenchChem are specifically designed for in-vitro studies, which are conducted outside of living organisms. In-vitro studies, derived from the Latin term "in glass," involve experiments performed in controlled laboratory settings using cells or tissues. It is important to note that these products are not categorized as medicines or drugs, and they have not received approval from the FDA for the prevention, treatment, or cure of any medical condition, ailment, or disease. We must emphasize that any form of bodily introduction of these products into humans or animals is strictly prohibited by law. It is essential to adhere to these guidelines to ensure compliance with legal and ethical standards in research and experimentation.