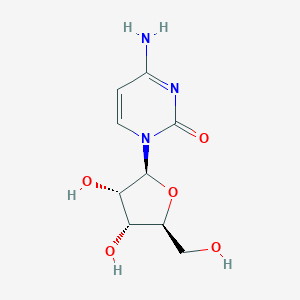
L-Cytidine
Overview
Description
L-Cytidine is a complex organic compound with significant importance in various scientific fields. This compound is characterized by its unique structure, which includes a pyrimidine ring attached to a tetrahydrofuran ring with multiple hydroxyl groups. Its molecular formula is C9H13N3O5.
Mechanism of Action
Target of Action
L-Cytidine primarily targets the enzyme Uridine-cytidine kinase 2 . This enzyme plays a crucial role in the pyrimidine salvage pathway, which is essential for nucleotide synthesis .
Mode of Action
As an analogue of cytidine, this compound can incorporate into RNA and DNA, disrupting RNA metabolism and inhibiting protein and DNA synthesis . It can also inhibit DNA methyltransferase, impairing DNA methylation .
Biochemical Pathways
This compound affects the pyrimidine salvage pathway, which is responsible for the recycling of pyrimidines to synthesize nucleotides . It also impacts the de novo pathway of nucleotide synthesis .
Pharmacokinetics
It is known that its absorption, distribution, metabolism, and excretion (adme) properties significantly impact its bioavailability .
Result of Action
The incorporation of this compound into RNA and DNA and its inhibition of DNA methylation can lead to changes in gene expression . This can have various molecular and cellular effects, including the management of neuropsychiatric deficits associated with cerebrovascular diseases when used in combination with uridine .
Action Environment
The action of this compound can be influenced by various environmental factors. For instance, adverse cellular conditions such as chronic inflammation can lead to its deregulation and overexpression . This can have significant implications for its action, efficacy, and stability.
Biochemical Analysis
Biochemical Properties
L-Cytidine interacts with several enzymes, proteins, and other biomolecules. For instance, it is commonly inactivated by cytidine deaminase (CDD), or by deoxycytidine monophosphate deaminase (dCMP deaminase) . Additional metabolic pathways, such as phosphorylation, can substantially contribute to its activation or inactivation .
Cellular Effects
This compound has significant effects on various types of cells and cellular processes. It influences cell function, including impact on cell signaling pathways, gene expression, and cellular metabolism . For instance, cytidine deaminase APOBEC3A has been found to promote cell growth and ribosome biogenesis .
Molecular Mechanism
At the molecular level, this compound exerts its effects through various mechanisms. For instance, it is involved in the formation of CDP-choline, a major brain phospholipid . It also serves as a choline donor in the biosynthesis of the neurotransmitter acetylcholine .
Temporal Effects in Laboratory Settings
In laboratory settings, the effects of this compound change over time. For instance, in a study involving E. coli, it was found that overexpression of the heterologous pyrP and nupC genes increased the expression levels of uracil permease and nucleoside transporter, and cytidine production by E. coli was greatly improved .
Dosage Effects in Animal Models
The effects of this compound vary with different dosages in animal models. For instance, it has been found that the median lethal dose (LD50) of IV citicoline in animal models is approximately 44 times higher than that of an equivalent IV dose of choline .
Metabolic Pathways
This compound is involved in several metabolic pathways. For instance, it is a key intermediate in the synthesis of phosphatidylcholine, a major brain phospholipid . It also serves as a choline donor in the biosynthesis of the neurotransmitter acetylcholine .
Transport and Distribution
This compound is transported and distributed within cells and tissues. For instance, it has been found that overexpression of the heterologous pyrP and nupC genes in E. coli increased the expression levels of uracil permease and nucleoside transporter, thereby enhancing the secretion of cytidine from E. coli .
Subcellular Localization
It is known that this compound is a component of RNA, suggesting that it is likely to be localized in the nucleus where RNA synthesis occurs .
Preparation Methods
Synthetic Routes and Reaction Conditions
The synthesis of L-Cytidine typically involves multiple steps, starting from readily available precursors. One common method involves the condensation of a suitable pyrimidine derivative with a protected sugar moiety, followed by deprotection and purification steps. The reaction conditions often require controlled temperatures, specific solvents, and catalysts to ensure high yield and purity.
Industrial Production Methods
In an industrial setting, the production of this compound may involve large-scale batch reactions with optimized conditions to maximize efficiency and minimize costs. The use of automated reactors and continuous flow systems can enhance the scalability of the synthesis process. Quality control measures, such as high-performance liquid chromatography (HPLC), are employed to ensure the consistency and quality of the final product.
Chemical Reactions Analysis
Types of Reactions
L-Cytidine undergoes various chemical reactions, including:
Oxidation: The hydroxyl groups can be oxidized to form corresponding ketones or aldehydes.
Reduction: The compound can be reduced to modify the functional groups, such as converting nitro groups to amines.
Substitution: The amino group can participate in nucleophilic substitution reactions, leading to the formation of various derivatives.
Common Reagents and Conditions
Common reagents used in these reactions include oxidizing agents like potassium permanganate, reducing agents like sodium borohydride, and nucleophiles for substitution reactions. The reaction conditions vary depending on the desired transformation, but typically involve controlled temperatures, specific solvents, and catalysts.
Major Products
The major products formed from these reactions depend on the specific reagents and conditions used. For example, oxidation of the hydroxyl groups can yield ketones or aldehydes, while substitution reactions can produce a wide range of derivatives with different functional groups.
Scientific Research Applications
L-Cytidine has numerous applications in scientific research, including:
Chemistry: Used as a building block for the synthesis of more complex molecules.
Biology: Studied for its potential role in biochemical pathways and interactions with biomolecules.
Medicine: Investigated for its therapeutic potential in treating various diseases, including cancer and viral infections.
Industry: Utilized in the development of new materials and chemical processes.
Comparison with Similar Compounds
Similar Compounds
Cytosine: A pyrimidine base found in DNA and RNA, similar in structure but lacking the tetrahydrofuran ring.
Uracil: Another pyrimidine base in RNA, also lacking the tetrahydrofuran ring.
Thymine: A pyrimidine base in DNA, with a structure similar to uracil but with a methyl group.
Uniqueness
L-Cytidine is unique due to its combination of a pyrimidine ring with a tetrahydrofuran ring, which imparts distinct chemical and biological properties. This structural uniqueness allows it to participate in specific reactions and interactions that are not possible with simpler pyrimidine derivatives.
Properties
IUPAC Name |
4-amino-1-[(2S,3S,4R,5S)-3,4-dihydroxy-5-(hydroxymethyl)oxolan-2-yl]pyrimidin-2-one | |
---|---|---|
Source | PubChem | |
URL | https://pubchem.ncbi.nlm.nih.gov | |
Description | Data deposited in or computed by PubChem | |
InChI |
InChI=1S/C9H13N3O5/c10-5-1-2-12(9(16)11-5)8-7(15)6(14)4(3-13)17-8/h1-2,4,6-8,13-15H,3H2,(H2,10,11,16)/t4-,6-,7-,8-/m0/s1 | |
Source | PubChem | |
URL | https://pubchem.ncbi.nlm.nih.gov | |
Description | Data deposited in or computed by PubChem | |
InChI Key |
UHDGCWIWMRVCDJ-PSQAKQOGSA-N | |
Source | PubChem | |
URL | https://pubchem.ncbi.nlm.nih.gov | |
Description | Data deposited in or computed by PubChem | |
Canonical SMILES |
C1=CN(C(=O)N=C1N)C2C(C(C(O2)CO)O)O | |
Source | PubChem | |
URL | https://pubchem.ncbi.nlm.nih.gov | |
Description | Data deposited in or computed by PubChem | |
Isomeric SMILES |
C1=CN(C(=O)N=C1N)[C@@H]2[C@H]([C@H]([C@@H](O2)CO)O)O | |
Source | PubChem | |
URL | https://pubchem.ncbi.nlm.nih.gov | |
Description | Data deposited in or computed by PubChem | |
Molecular Formula |
C9H13N3O5 | |
Source | PubChem | |
URL | https://pubchem.ncbi.nlm.nih.gov | |
Description | Data deposited in or computed by PubChem | |
DSSTOX Substance ID |
DTXSID40276262 | |
Record name | L-Cytidine | |
Source | EPA DSSTox | |
URL | https://comptox.epa.gov/dashboard/DTXSID40276262 | |
Description | DSSTox provides a high quality public chemistry resource for supporting improved predictive toxicology. | |
Molecular Weight |
243.22 g/mol | |
Source | PubChem | |
URL | https://pubchem.ncbi.nlm.nih.gov | |
Description | Data deposited in or computed by PubChem | |
CAS No. |
26524-60-7 | |
Record name | L-Cytidine | |
Source | EPA DSSTox | |
URL | https://comptox.epa.gov/dashboard/DTXSID40276262 | |
Description | DSSTox provides a high quality public chemistry resource for supporting improved predictive toxicology. | |
Retrosynthesis Analysis
AI-Powered Synthesis Planning: Our tool employs the Template_relevance Pistachio, Template_relevance Bkms_metabolic, Template_relevance Pistachio_ringbreaker, Template_relevance Reaxys, Template_relevance Reaxys_biocatalysis model, leveraging a vast database of chemical reactions to predict feasible synthetic routes.
One-Step Synthesis Focus: Specifically designed for one-step synthesis, it provides concise and direct routes for your target compounds, streamlining the synthesis process.
Accurate Predictions: Utilizing the extensive PISTACHIO, BKMS_METABOLIC, PISTACHIO_RINGBREAKER, REAXYS, REAXYS_BIOCATALYSIS database, our tool offers high-accuracy predictions, reflecting the latest in chemical research and data.
Strategy Settings
Precursor scoring | Relevance Heuristic |
---|---|
Min. plausibility | 0.01 |
Model | Template_relevance |
Template Set | Pistachio/Bkms_metabolic/Pistachio_ringbreaker/Reaxys/Reaxys_biocatalysis |
Top-N result to add to graph | 6 |
Feasible Synthetic Routes
Q1: What makes L-Cytidine a potential antiviral agent?
A1: Unlike its naturally occurring D-enantiomer, this compound demonstrates potent antiviral activity against human immunodeficiency virus type 1 (HIV-1) and hepatitis B virus (HBV) in vitro. [, , ] This is attributed to its unique interaction with viral enzymes, inhibiting their replication cycle. [, ]
Q2: How does this compound compare to its D-enantiomer (D-Cytidine) in terms of antiviral activity?
A2: this compound exhibits significantly higher potency against HBV compared to D-Cytidine. Specifically, this compound is approximately 1000 times more potent than its D-counterpart, 2',3'-dideoxy-beta-D-5-fluorocytidine (beta-D-FddC). [] This difference highlights the importance of stereochemistry in antiviral drug design.
Q3: How does this compound exert its antiviral effects against HIV-1 and HBV?
A3: While the precise mechanism of action is still under investigation, this compound likely acts as a chain terminator during viral DNA synthesis. [, ] It's suggested that this compound is phosphorylated within the cell to its active triphosphate form, which then competes with natural nucleotides for incorporation into the growing viral DNA chain, ultimately halting further elongation.
Q4: Does this compound show any activity against other viruses?
A4: Research has shown that this compound and its 5-fluoro derivative, 2′,3′-Dideoxy-β-L-5-fluorocytidine (β-L-FddC), do not exhibit activity against Herpes Simplex Viruses 1 and 2. [] This suggests a degree of specificity towards HIV-1 and HBV.
Q5: Are there any known toxicities associated with this compound?
A5: While this compound shows a favorable toxicity profile compared to ddC, it has exhibited in vitro cellular toxicity in several leukocytic cell lines. [] Additionally, it has been shown to inhibit phytohemagglutinin-stimulated human peripheral blood mononuclear leukocyte proliferation, suggesting potential immunomodulatory effects. [, ] Further research is needed to fully elucidate its safety profile in vivo.
Q6: Has this compound been tested in clinical trials?
A6: To date, there are no published reports of clinical trials involving this compound. Further preclinical studies are crucial to assess its efficacy and safety before advancing to human trials.
Q7: What is the molecular formula and weight of this compound?
A7: The molecular formula of this compound is C9H13N3O5, and its molecular weight is 243.22 g/mol.
Q8: What is the impact of structural modifications on the activity of this compound?
A8: Research suggests that even minor modifications to the this compound structure can significantly impact its antiviral activity and cytotoxicity. For instance, the addition of a fluorine atom at the 5-position of the cytosine base, creating β-L-FddC, leads to enhanced anti-HIV activity compared to the unmodified this compound. [, ] Conversely, the 4'-thio-L-nucleosides, incorporating a sulfur atom in the ribose ring, did not exhibit antiviral activity against HIV or HBV. [] This highlights the importance of exploring structure-activity relationships (SAR) to optimize the antiviral potency and safety of this compound derivatives.
Q9: Have any prodrugs of this compound been developed?
A9: Yes, to enhance the oral bioavailability of this compound, researchers have synthesized various prodrugs, such as the 3′-mono-, 5′-mono-, and 3′,5′-di-O-valinyl esters. [] Among these, the 3′-mono-O-valinyl derivative has shown promise as a potential anti-HBV agent due to its favorable pharmacokinetic profile in cynomolgus monkeys. []
Disclaimer and Information on In-Vitro Research Products
Please be aware that all articles and product information presented on BenchChem are intended solely for informational purposes. The products available for purchase on BenchChem are specifically designed for in-vitro studies, which are conducted outside of living organisms. In-vitro studies, derived from the Latin term "in glass," involve experiments performed in controlled laboratory settings using cells or tissues. It is important to note that these products are not categorized as medicines or drugs, and they have not received approval from the FDA for the prevention, treatment, or cure of any medical condition, ailment, or disease. We must emphasize that any form of bodily introduction of these products into humans or animals is strictly prohibited by law. It is essential to adhere to these guidelines to ensure compliance with legal and ethical standards in research and experimentation.