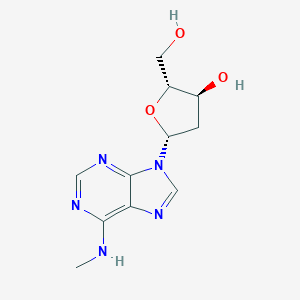
N6-Methyl-2'-deoxyadenosine
Overview
Description
N6-Methyl-2’-deoxyadenosine is a modified nucleoside that plays a significant role in various biological processes. It is a derivative of deoxyadenosine, where a methyl group is added to the nitrogen at the sixth position of the adenine ring. This modification is known to influence gene expression and is involved in epigenetic regulation .
Mechanism of Action
Target of Action
N6-Methyl-2’-deoxyadenosine (N6-Me-dAdo) is a DNA modification that primarily targets the DNA itself . It is a prevalent DNA modification in prokaryotes and has recently been identified in higher eukaryotes, including mammals . It is located at the transcription start site and plays a role in increased gene expression .
Mode of Action
N6-Me-dAdo interacts with its targets by accumulating along promoters and coding sequences in activated cells . The deposition of N6-Me-dAdo is associated with increased genome-wide occupancy of the mammalian N6-Me-dAdo methyltransferase, N6amt1 . This correlates with induced gene expression . The accumulation of N6-Me-dAdo is associated with transcriptional activation at specific promoters, which is required for messenger RNA expression .
Biochemical Pathways
N6-Me-dAdo is involved in the regulation of gene transcription, DNA repair, and replication in prokaryotes and protists . It is also involved in the regulation of experience-dependent gene expression in the adult brain . Methyltransferase METTL4 or N6AMT1 catalyze the transfer of a methyl group from the methyl donor S-adenosylmethionine (SAM) to unmethylated A, leading to a 6mA site and S-adenosylhomocysteine (SAH) . 6mA can be oxidatively demethylated by ALKBH1 and ALKBH4 using oxygen, alpha-ketoglutarate (α-KG), and ferrous ion (Fe 2+), leading to an unstable intermediate .
Pharmacokinetics
It is known that n6-me-dado is a precursor of n6-methyl-2’-deoxyadenosine 3’, 5’-bisphosphate (n6mabp), a competitive antagonist specific to the p2y1 receptors in the p2y receptor family .
Result of Action
The molecular and cellular effects of N6-Me-dAdo’s action include the promotion of hyperproliferation of certain progenitor cells and the repression of apoptosis of erythroid progenitor cells (EPCs) . It also drives activity-induced gene expression and is required for fear extinction .
Action Environment
The action of N6-Me-dAdo is influenced by environmental factors such as neuronal activation . In adult mice trained in fear extinction, N6-Me-dAdo accumulates along promoters and coding sequences in activated prefrontal cortical neurons . This highlights changes in N6-Me-dAdo as an epigenetic mechanism associated with activity-induced gene expression and the formation of fear extinction memory .
Biochemical Analysis
Biochemical Properties
N6-Methyl-2’-deoxyadenosine interacts with several enzymes and proteins. Methyltransferase METTL4 or N6AMT1 catalyzes the transfer of a methyl group from the methyl donor S-adenosylmethionine (SAM) to unmethylated adenine, leading to a N6-Methyl-2’-deoxyadenosine site and S-adenosylhomocysteine (SAH) . N6-Methyl-2’-deoxyadenosine can be oxidatively demethylated by ALKBH1 and ALKBH4 using oxygen, alpha-ketoglutarate (α-KG), and ferrous ion (Fe 2+), leading to an unstable intermediate .
Cellular Effects
N6-Methyl-2’-deoxyadenosine has significant effects on various types of cells and cellular processes. It influences cell function by impacting cell signaling pathways, gene expression, and cellular metabolism . For instance, N6-Methyl-2’-deoxyadenosine promotes the hyperproliferation of burst-forming unit erythroid (BFU-E) progenitor cells and represses the apoptosis of erythroid progenitor cells (EPCs) .
Molecular Mechanism
N6-Methyl-2’-deoxyadenosine exerts its effects at the molecular level through binding interactions with biomolecules, enzyme inhibition or activation, and changes in gene expression . For example, N6-Methyl-2’-deoxyadenosine enhances and prolongs the activation of erythropoiesis-associated master gene c-Kit and its downstream signaling, leading to expansion and accumulation of EPCs .
Temporal Effects in Laboratory Settings
The effects of N6-Methyl-2’-deoxyadenosine change over time in laboratory settings. It has been observed that N6-Methyl-2’-deoxyadenosine stimulates the self-renewal of BFU-E progenitor cells and enhances the activation of c-Kit signaling pathways .
Metabolic Pathways
N6-Methyl-2’-deoxyadenosine is involved in several metabolic pathways. It interacts with enzymes such as METTL4 or N6AMT1 and cofactors like SAM
Subcellular Localization
It is believed that N6-Methyl-2’-deoxyadenosine may be directed to specific compartments or organelles through targeting signals or post-translational modifications .
Preparation Methods
Synthetic Routes and Reaction Conditions: The synthesis of N6-Methyl-2’-deoxyadenosine typically involves the methylation of deoxyadenosine. One common method includes the use of methyl iodide as the methylating agent in the presence of a base such as sodium hydride. The reaction is carried out in an aprotic solvent like dimethylformamide at elevated temperatures to facilitate the methylation process .
Industrial Production Methods: Industrial production of N6-Methyl-2’-deoxyadenosine may involve more scalable and efficient methods, such as enzymatic methylation using specific methyltransferases. These enzymes catalyze the transfer of a methyl group from S-adenosylmethionine to deoxyadenosine, producing N6-Methyl-2’-deoxyadenosine with high specificity and yield .
Chemical Reactions Analysis
Types of Reactions: N6-Methyl-2’-deoxyadenosine can undergo various chemical reactions, including:
Oxidation: This reaction can be facilitated by oxidizing agents like hydrogen peroxide, leading to the formation of oxidized derivatives.
Reduction: Reduction reactions can be carried out using reducing agents such as sodium borohydride.
Substitution: Nucleophilic substitution reactions can occur at the methyl group, where nucleophiles like thiols or amines replace the methyl group.
Common Reagents and Conditions:
Oxidation: Hydrogen peroxide in aqueous solution.
Reduction: Sodium borohydride in methanol.
Substitution: Thiols or amines in the presence of a base like sodium hydroxide.
Major Products:
Oxidation: Oxidized derivatives of N6-Methyl-2’-deoxyadenosine.
Reduction: Reduced forms of the compound.
Substitution: Substituted derivatives where the methyl group is replaced by other functional groups.
Scientific Research Applications
N6-Methyl-2’-deoxyadenosine has a wide range of applications in scientific research:
Biology: Plays a role in gene expression regulation and epigenetic modifications.
Industry: Utilized in the production of specific inhibitors for biochemical studies and drug development.
Comparison with Similar Compounds
N6-Methyladenosine: Similar to N6-Methyl-2’-deoxyadenosine but lacks the deoxyribose sugar.
5-Methylcytosine: Another epigenetic marker involved in gene regulation.
N4-Methylcytosine: Found in prokaryotes and involved in DNA protection and gene regulation.
Uniqueness: N6-Methyl-2’-deoxyadenosine is unique due to its specific role in the regulation of gene expression in eukaryotic cells. Unlike other methylated nucleosides, it is specifically involved in the methylation of adenine in DNA, which has distinct biological implications .
Properties
IUPAC Name |
(2R,3S,5R)-2-(hydroxymethyl)-5-[6-(methylamino)purin-9-yl]oxolan-3-ol | |
---|---|---|
Source | PubChem | |
URL | https://pubchem.ncbi.nlm.nih.gov | |
Description | Data deposited in or computed by PubChem | |
InChI |
InChI=1S/C11H15N5O3/c1-12-10-9-11(14-4-13-10)16(5-15-9)8-2-6(18)7(3-17)19-8/h4-8,17-18H,2-3H2,1H3,(H,12,13,14)/t6-,7+,8+/m0/s1 | |
Source | PubChem | |
URL | https://pubchem.ncbi.nlm.nih.gov | |
Description | Data deposited in or computed by PubChem | |
InChI Key |
DYSDOYRQWBDGQQ-XLPZGREQSA-N | |
Source | PubChem | |
URL | https://pubchem.ncbi.nlm.nih.gov | |
Description | Data deposited in or computed by PubChem | |
Canonical SMILES |
CNC1=C2C(=NC=N1)N(C=N2)C3CC(C(O3)CO)O | |
Source | PubChem | |
URL | https://pubchem.ncbi.nlm.nih.gov | |
Description | Data deposited in or computed by PubChem | |
Isomeric SMILES |
CNC1=C2C(=NC=N1)N(C=N2)[C@H]3C[C@@H]([C@H](O3)CO)O | |
Source | PubChem | |
URL | https://pubchem.ncbi.nlm.nih.gov | |
Description | Data deposited in or computed by PubChem | |
Molecular Formula |
C11H15N5O3 | |
Source | PubChem | |
URL | https://pubchem.ncbi.nlm.nih.gov | |
Description | Data deposited in or computed by PubChem | |
DSSTOX Substance ID |
DTXSID60173840 | |
Record name | N(6)-Methyl-2'-deoxyadenosine | |
Source | EPA DSSTox | |
URL | https://comptox.epa.gov/dashboard/DTXSID60173840 | |
Description | DSSTox provides a high quality public chemistry resource for supporting improved predictive toxicology. | |
Molecular Weight |
265.27 g/mol | |
Source | PubChem | |
URL | https://pubchem.ncbi.nlm.nih.gov | |
Description | Data deposited in or computed by PubChem | |
CAS No. |
2002-35-9 | |
Record name | 2′-Deoxy-N6-methyladenosine | |
Source | CAS Common Chemistry | |
URL | https://commonchemistry.cas.org/detail?cas_rn=2002-35-9 | |
Description | CAS Common Chemistry is an open community resource for accessing chemical information. Nearly 500,000 chemical substances from CAS REGISTRY cover areas of community interest, including common and frequently regulated chemicals, and those relevant to high school and undergraduate chemistry classes. This chemical information, curated by our expert scientists, is provided in alignment with our mission as a division of the American Chemical Society. | |
Explanation | The data from CAS Common Chemistry is provided under a CC-BY-NC 4.0 license, unless otherwise stated. | |
Record name | N(6)-Methyl-2'-deoxyadenosine | |
Source | ChemIDplus | |
URL | https://pubchem.ncbi.nlm.nih.gov/substance/?source=chemidplus&sourceid=0002002359 | |
Description | ChemIDplus is a free, web search system that provides access to the structure and nomenclature authority files used for the identification of chemical substances cited in National Library of Medicine (NLM) databases, including the TOXNET system. | |
Record name | N(6)-Methyl-2'-deoxyadenosine | |
Source | EPA DSSTox | |
URL | https://comptox.epa.gov/dashboard/DTXSID60173840 | |
Description | DSSTox provides a high quality public chemistry resource for supporting improved predictive toxicology. | |
Record name | N6-METHYLDEOXYADENOSINE | |
Source | FDA Global Substance Registration System (GSRS) | |
URL | https://gsrs.ncats.nih.gov/ginas/app/beta/substances/48QZW2IR9H | |
Description | The FDA Global Substance Registration System (GSRS) enables the efficient and accurate exchange of information on what substances are in regulated products. Instead of relying on names, which vary across regulatory domains, countries, and regions, the GSRS knowledge base makes it possible for substances to be defined by standardized, scientific descriptions. | |
Explanation | Unless otherwise noted, the contents of the FDA website (www.fda.gov), both text and graphics, are not copyrighted. They are in the public domain and may be republished, reprinted and otherwise used freely by anyone without the need to obtain permission from FDA. Credit to the U.S. Food and Drug Administration as the source is appreciated but not required. | |
Retrosynthesis Analysis
AI-Powered Synthesis Planning: Our tool employs the Template_relevance Pistachio, Template_relevance Bkms_metabolic, Template_relevance Pistachio_ringbreaker, Template_relevance Reaxys, Template_relevance Reaxys_biocatalysis model, leveraging a vast database of chemical reactions to predict feasible synthetic routes.
One-Step Synthesis Focus: Specifically designed for one-step synthesis, it provides concise and direct routes for your target compounds, streamlining the synthesis process.
Accurate Predictions: Utilizing the extensive PISTACHIO, BKMS_METABOLIC, PISTACHIO_RINGBREAKER, REAXYS, REAXYS_BIOCATALYSIS database, our tool offers high-accuracy predictions, reflecting the latest in chemical research and data.
Strategy Settings
Precursor scoring | Relevance Heuristic |
---|---|
Min. plausibility | 0.01 |
Model | Template_relevance |
Template Set | Pistachio/Bkms_metabolic/Pistachio_ringbreaker/Reaxys/Reaxys_biocatalysis |
Top-N result to add to graph | 6 |
Feasible Synthetic Routes
Q1: What is the significance of N6-Methyl-2'-deoxyadenosine (m6dA) as an epigenetic mark?
A1: Unlike genetic information encoded in the DNA sequence itself, epigenetic marks like m6dA represent modifications that influence gene expression without altering the underlying sequence. These marks play a crucial role in regulating cellular processes, development, and disease.
Q2: Is there a link between m6dA and fear extinction memory?
A2: Emerging research suggests a connection between m6dA and fear extinction memory. Studies in mice have shown that m6dA levels increase in the prefrontal cortex, a brain region crucial for memory and learning, during fear extinction learning [, ]. This accumulation of m6dA appears necessary for the proper expression of genes, such as bdnf, involved in forming fear extinction memories.
Q3: Can environmental factors influence m6dA levels?
A3: While research is ongoing, studies indicate that environmental contaminants might influence m6dA levels. For instance, research on amphipods suggests a link between exposure to environmental pollutants, alterations in m6dA levels, and the occurrence of embryo malformations [, ]. This suggests that environmental factors might disrupt epigenetic regulation via m6dA, potentially impacting development and potentially contributing to disease susceptibility.
Q4: Are there connections between m6dA and cancer?
A4: While research is in its early stages, some studies suggest a potential link between m6dA and cancer. For example, researchers observed significantly elevated levels of hm6dA, a hydroxylated derivative of m6dA, in lung carcinoma tissues []. This increase is thought to be related to the higher expression of the ALKBH1 protein, which facilitates hm6dA formation from m6dA. The exact role of hm6dA in cancer development remains to be determined.
Q5: What are the common methods used to measure m6dA levels?
A5: High-performance liquid chromatography coupled with tandem mass spectrometry (LC-MS/MS) is a widely used technique for quantifying m6dA levels in various biological samples [, , , ]. This method offers high sensitivity and specificity, allowing for accurate measurement of this relatively low-abundance DNA modification.
Q6: Beyond measuring overall m6dA levels, can we determine its distribution within the genome?
A6: Yes, techniques like m6dA-sequencing (m6dA-seq) can map the distribution of m6dA across the genome. This approach provides insights into the genomic regions where m6dA is enriched and its potential regulatory roles at specific gene loci.
Q7: What challenges are associated with studying m6dA, and how are researchers addressing them?
A7: One of the primary challenges in studying m6dA is its relatively low abundance in DNA compared to other modifications like 5-methylcytosine. This necessitates highly sensitive and specific detection methods, such as LC-MS/MS and m6dA-seq. Furthermore, distinguishing genuine m6dA signals from background noise or contamination is crucial, leading to the development of improved methods and controls [].
Disclaimer and Information on In-Vitro Research Products
Please be aware that all articles and product information presented on BenchChem are intended solely for informational purposes. The products available for purchase on BenchChem are specifically designed for in-vitro studies, which are conducted outside of living organisms. In-vitro studies, derived from the Latin term "in glass," involve experiments performed in controlled laboratory settings using cells or tissues. It is important to note that these products are not categorized as medicines or drugs, and they have not received approval from the FDA for the prevention, treatment, or cure of any medical condition, ailment, or disease. We must emphasize that any form of bodily introduction of these products into humans or animals is strictly prohibited by law. It is essential to adhere to these guidelines to ensure compliance with legal and ethical standards in research and experimentation.