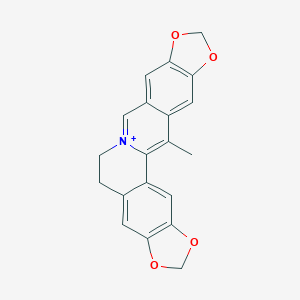
Worenine
Overview
Description
Scientific Research Applications
Worenin has several scientific research applications, particularly in the fields of chemistry, biology, and medicine. In chemistry, it is used as a model compound to study reaction mechanisms and the behavior of peroxisome-derived vesicles. In biology, Worenin is crucial for understanding the function of Woronin bodies in filamentous fungi, which play a role in cellular damage response and nutrient stress survival . In medicine, research on Worenin and its derivatives may lead to the development of new therapeutic agents targeting fungal infections and other diseases .
Mechanism of Action
Target of Action
Worenine, an isoquinoline alkaloid isolated from Coptis chinensis, primarily targets Hypoxia-inducible factor 1-alpha (HIF-1α) . HIF-1α is a transcription factor that plays a crucial role in cellular responses to hypoxia, including the regulation of energy metabolism, angiogenesis, and cell survival . In addition, this compound has been found to inhibit JNK2 , a protein kinase involved in various cellular processes such as inflammation, apoptosis, and cellular growth .
Mode of Action
This compound interacts with its targets, leading to significant changes in cellular processes. It negatively regulates HIF-1α, thereby inhibiting the growth of colorectal cancer cells . This compound also inhibits the JNK-ATF2/c-jun signaling pathway, which plays a role in cellular stress responses .
Biochemical Pathways
This compound affects several biochemical pathways. It alters glucose metabolism and glycolysis, a phenomenon known as the Warburg effect . The Warburg effect is a metabolic reprogramming that occurs in cancer cells, where they preferentially utilize glycolysis over oxidative phosphorylation for energy production, even under normoxic conditions . By reversing this effect, this compound can inhibit the growth of cancer cells .
Pharmacokinetics
It is known that this compound is soluble in methanol, ethanol, and dmso
Result of Action
This compound’s action results in the inhibition of colorectal cancer cell growth, proliferation, and cell cycle progression . It also impairs the Warburg effect, altering the glucose metabolism of cancer cells . In addition, this compound has been found to prevent solar ultraviolet-induced sunburn by inhibiting JNK2 .
Action Environment
The environment can influence the action, efficacy, and stability of this compound. For instance, the compound’s solubility in various solvents suggests that its action might be influenced by the cellular and extracellular environment . .
Biochemical Analysis
Biochemical Properties
Worenine plays a significant role in biochemical reactions, particularly in the context of cancer cell metabolism. It interacts with various enzymes and proteins involved in glycolysis, such as phosphofructokinase (PFK-L), hexokinase 2 (HK2), and pyruvate kinase M2 (PKM2). These interactions lead to alterations in glucose uptake, consumption, and lactate production, ultimately affecting the glycolytic pathway . This compound’s ability to inhibit hypoxia-inducible factor 1-alpha (HIF-1α) further underscores its impact on cellular metabolism and cancer cell proliferation .
Cellular Effects
This compound exerts profound effects on various cell types and cellular processes. In colorectal cancer cells, it has been shown to inhibit cell proliferation, colony formation, and cell cycle progression. This compound’s influence extends to cell signaling pathways, where it negatively regulates HIF-1α, leading to reduced glycolysis and altered glucose metabolism . Additionally, this compound impacts gene expression by modulating the activity of glycolytic enzymes and other related proteins .
Molecular Mechanism
At the molecular level, this compound exerts its effects through several mechanisms. It binds to and inhibits HIF-1α, a key regulator of cellular response to hypoxia. This inhibition leads to decreased expression of glycolytic enzymes and reduced glycolysis . This compound also affects enzyme activity by interacting with PFK-L, HK2, and PKM2, further disrupting the glycolytic pathway . These molecular interactions contribute to this compound’s anti-cancer properties and its ability to reverse the Warburg effect .
Temporal Effects in Laboratory Settings
In laboratory settings, the effects of this compound have been observed to change over time. Studies have shown that this compound remains stable under various conditions, maintaining its inhibitory effects on cancer cell proliferation and glycolysis . Long-term exposure to this compound has demonstrated sustained anti-cancer activity, with minimal degradation observed in in vitro and in vivo studies .
Dosage Effects in Animal Models
The effects of this compound vary with different dosages in animal models. At lower doses, this compound effectively inhibits cancer cell growth and proliferation without causing significant toxicity . At higher doses, some adverse effects have been observed, including potential toxicity and negative impacts on normal cellular functions . These findings highlight the importance of determining optimal dosage levels for therapeutic applications.
Metabolic Pathways
This compound is involved in several metabolic pathways, primarily affecting glycolysis. It interacts with enzymes such as PFK-L, HK2, and PKM2, leading to alterations in glucose metabolism and lactate production . This compound’s inhibition of HIF-1α further impacts metabolic flux and metabolite levels, contributing to its anti-cancer effects .
Transport and Distribution
Within cells and tissues, this compound is transported and distributed through various mechanisms. It interacts with transporters and binding proteins that facilitate its localization and accumulation in specific cellular compartments . These interactions play a crucial role in this compound’s ability to exert its effects on cellular metabolism and cancer cell proliferation .
Subcellular Localization
This compound’s subcellular localization is essential for its activity and function. It is directed to specific compartments and organelles through targeting signals and post-translational modifications . This localization allows this compound to effectively interact with its target enzymes and proteins, thereby modulating cellular processes and exerting its anti-cancer effects .
Preparation Methods
The preparation of Worenin involves several synthetic routes and reaction conditions. While specific details on the synthesis of Worenin itself are limited, the general approach involves the use of organic synthesis techniques. Industrial production methods for similar compounds often involve multi-step synthesis processes that include the formation of intermediate compounds, purification, and final product isolation .
Chemical Reactions Analysis
Worenin undergoes various chemical reactions, including oxidation, reduction, and substitution. Common reagents used in these reactions include oxidizing agents, reducing agents, and nucleophiles. The major products formed from these reactions depend on the specific conditions and reagents used. For example, oxidation reactions may yield oxidized derivatives, while substitution reactions can result in the formation of new functional groups on the Worenin molecule .
Comparison with Similar Compounds
Worenin can be compared with other similar compounds, such as other peroxisome-derived vesicles found in different fungal species. What sets Worenin apart is its specific role in the formation of Woronin bodies and its unique molecular structure. Similar compounds include Hex1 protein derivatives and other peroxisome-associated vesicles .
Properties
IUPAC Name |
24-methyl-5,7,18,20-tetraoxa-13-azoniahexacyclo[11.11.0.02,10.04,8.015,23.017,21]tetracosa-1(24),2,4(8),9,13,15,17(21),22-octaene | |
---|---|---|
Source | PubChem | |
URL | https://pubchem.ncbi.nlm.nih.gov | |
Description | Data deposited in or computed by PubChem | |
InChI |
InChI=1S/C20H16NO4/c1-11-14-6-18-17(23-10-24-18)5-13(14)8-21-3-2-12-4-16-19(25-9-22-16)7-15(12)20(11)21/h4-8H,2-3,9-10H2,1H3/q+1 | |
Source | PubChem | |
URL | https://pubchem.ncbi.nlm.nih.gov | |
Description | Data deposited in or computed by PubChem | |
InChI Key |
LCXREBMNASQAOC-UHFFFAOYSA-N | |
Source | PubChem | |
URL | https://pubchem.ncbi.nlm.nih.gov | |
Description | Data deposited in or computed by PubChem | |
Canonical SMILES |
CC1=C2C3=CC4=C(C=C3CC[N+]2=CC5=CC6=C(C=C15)OCO6)OCO4 | |
Source | PubChem | |
URL | https://pubchem.ncbi.nlm.nih.gov | |
Description | Data deposited in or computed by PubChem | |
Molecular Formula |
C20H16NO4+ | |
Source | PubChem | |
URL | https://pubchem.ncbi.nlm.nih.gov | |
Description | Data deposited in or computed by PubChem | |
Molecular Weight |
334.3 g/mol | |
Source | PubChem | |
URL | https://pubchem.ncbi.nlm.nih.gov | |
Description | Data deposited in or computed by PubChem | |
CAS No. |
38763-29-0 | |
Record name | Worenine ion | |
Source | ChemIDplus | |
URL | https://pubchem.ncbi.nlm.nih.gov/substance/?source=chemidplus&sourceid=0038763290 | |
Description | ChemIDplus is a free, web search system that provides access to the structure and nomenclature authority files used for the identification of chemical substances cited in National Library of Medicine (NLM) databases, including the TOXNET system. | |
Record name | WORENINE ION | |
Source | FDA Global Substance Registration System (GSRS) | |
URL | https://gsrs.ncats.nih.gov/ginas/app/beta/substances/STF4A6F4EH | |
Description | The FDA Global Substance Registration System (GSRS) enables the efficient and accurate exchange of information on what substances are in regulated products. Instead of relying on names, which vary across regulatory domains, countries, and regions, the GSRS knowledge base makes it possible for substances to be defined by standardized, scientific descriptions. | |
Explanation | Unless otherwise noted, the contents of the FDA website (www.fda.gov), both text and graphics, are not copyrighted. They are in the public domain and may be republished, reprinted and otherwise used freely by anyone without the need to obtain permission from FDA. Credit to the U.S. Food and Drug Administration as the source is appreciated but not required. | |
Retrosynthesis Analysis
AI-Powered Synthesis Planning: Our tool employs the Template_relevance Pistachio, Template_relevance Bkms_metabolic, Template_relevance Pistachio_ringbreaker, Template_relevance Reaxys, Template_relevance Reaxys_biocatalysis model, leveraging a vast database of chemical reactions to predict feasible synthetic routes.
One-Step Synthesis Focus: Specifically designed for one-step synthesis, it provides concise and direct routes for your target compounds, streamlining the synthesis process.
Accurate Predictions: Utilizing the extensive PISTACHIO, BKMS_METABOLIC, PISTACHIO_RINGBREAKER, REAXYS, REAXYS_BIOCATALYSIS database, our tool offers high-accuracy predictions, reflecting the latest in chemical research and data.
Strategy Settings
Precursor scoring | Relevance Heuristic |
---|---|
Min. plausibility | 0.01 |
Model | Template_relevance |
Template Set | Pistachio/Bkms_metabolic/Pistachio_ringbreaker/Reaxys/Reaxys_biocatalysis |
Top-N result to add to graph | 6 |
Feasible Synthetic Routes
Disclaimer and Information on In-Vitro Research Products
Please be aware that all articles and product information presented on BenchChem are intended solely for informational purposes. The products available for purchase on BenchChem are specifically designed for in-vitro studies, which are conducted outside of living organisms. In-vitro studies, derived from the Latin term "in glass," involve experiments performed in controlled laboratory settings using cells or tissues. It is important to note that these products are not categorized as medicines or drugs, and they have not received approval from the FDA for the prevention, treatment, or cure of any medical condition, ailment, or disease. We must emphasize that any form of bodily introduction of these products into humans or animals is strictly prohibited by law. It is essential to adhere to these guidelines to ensure compliance with legal and ethical standards in research and experimentation.