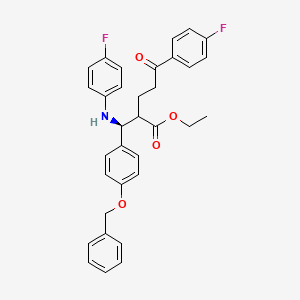
ethyl 2-((S)-(4-(benzyloxy)phenyl)((4-fluorophenyl)amino)methyl)-5-(4-fluorophenyl)-5-oxopentanoate
- Click on QUICK INQUIRY to receive a quote from our team of experts.
- With the quality product at a COMPETITIVE price, you can focus more on your research.
Overview
Description
Ethyl 2-((S)-(4-(benzyloxy)phenyl)((4-fluorophenyl)amino)methyl)-5-(4-fluorophenyl)-5-oxopentanoate is a complex organic compound that has garnered interest in various fields of scientific research. This compound is characterized by its unique structure, which includes multiple aromatic rings and functional groups, making it a versatile molecule for various chemical reactions and applications.
Preparation Methods
Synthetic Routes and Reaction Conditions
The synthesis of ethyl 2-((S)-(4-(benzyloxy)phenyl)((4-fluorophenyl)amino)methyl)-5-(4-fluorophenyl)-5-oxopentanoate typically involves multi-step organic synthesis. The process often starts with the preparation of key intermediates, such as benzyloxyphenyl and fluorophenyl derivatives. These intermediates are then subjected to various coupling reactions, including amination and esterification, under controlled conditions to form the final product.
Industrial Production Methods
Industrial production of this compound may involve large-scale synthesis using optimized reaction conditions to ensure high yield and purity. Techniques such as continuous flow reactors and automated synthesis platforms can be employed to streamline the production process and reduce costs.
Chemical Reactions Analysis
Types of Reactions
Ethyl 2-((S)-(4-(benzyloxy)phenyl)((4-fluorophenyl)amino)methyl)-5-(4-fluorophenyl)-5-oxopentanoate can undergo various types of chemical reactions, including:
Oxidation: The compound can be oxidized to form corresponding ketones or carboxylic acids.
Reduction: Reduction reactions can convert the compound into alcohols or amines.
Substitution: The aromatic rings in the compound can undergo electrophilic or nucleophilic substitution reactions.
Common Reagents and Conditions
Common reagents used in these reactions include oxidizing agents like potassium permanganate, reducing agents such as lithium aluminum hydride, and various nucleophiles and electrophiles for substitution reactions. Reaction conditions typically involve controlled temperatures, solvents, and catalysts to achieve the desired transformations.
Major Products
The major products formed from these reactions depend on the specific reaction conditions and reagents used. For example, oxidation may yield carboxylic acids, while reduction can produce alcohols or amines.
Scientific Research Applications
Ethyl 2-((S)-(4-(benzyloxy)phenyl)((4-fluorophenyl)amino)methyl)-5-(4-fluorophenyl)-5-oxopentanoate has several scientific research applications, including:
Chemistry: Used as a building block for the synthesis of more complex molecules.
Biology: Investigated for its potential biological activity and interactions with biomolecules.
Medicine: Explored for its potential therapeutic properties, including anti-inflammatory and anticancer activities.
Industry: Utilized in the development of new materials and chemical processes.
Mechanism of Action
The mechanism of action of ethyl 2-((S)-(4-(benzyloxy)phenyl)((4-fluorophenyl)amino)methyl)-5-(4-fluorophenyl)-5-oxopentanoate involves its interaction with specific molecular targets and pathways. The compound may bind to enzymes or receptors, modulating their activity and leading to various biological effects. The exact pathways and targets depend on the specific application and context of use.
Comparison with Similar Compounds
Ethyl 2-((S)-(4-(benzyloxy)phenyl)((4-fluorophenyl)amino)methyl)-5-(4-fluorophenyl)-5-oxopentanoate can be compared with other similar compounds, such as:
- Ethyl 2-((S)-(4-(benzyloxy)phenyl)((4-chlorophenyl)amino)methyl)-5-(4-chlorophenyl)-5-oxopentanoate
- Ethyl 2-((S)-(4-(benzyloxy)phenyl)((4-bromophenyl)amino)methyl)-5-(4-bromophenyl)-5-oxopentanoate
These compounds share similar structural features but differ in the substituents on the aromatic rings, which can influence their chemical reactivity and biological activity. The uniqueness of this compound lies in its specific combination of functional groups and their arrangement, which can result in distinct properties and applications.
Biological Activity
Ethyl 2-((S)-(4-(benzyloxy)phenyl)((4-fluorophenyl)amino)methyl)-5-(4-fluorophenyl)-5-oxopentanoate is a complex organic compound that has garnered interest in medicinal chemistry due to its potential biological activities. This article delves into its biological activity, synthesizing findings from various studies to present a comprehensive overview.
Chemical Structure and Properties
The compound is characterized by a unique structure that includes a benzyloxy group, fluorophenyl moieties, and an oxopentanoate backbone. Its molecular formula is C23H24F2N2O3, and it exhibits properties that may influence its biological interactions.
1. Inhibition of Enzymes
Recent studies have highlighted the compound's potential as an inhibitor of monoamine oxidase B (MAO-B), an enzyme implicated in neurodegenerative diseases such as Parkinson's disease. The compound demonstrated competitive inhibition with an IC50 value of 0.062 µM, indicating strong activity compared to established MAO-B inhibitors like rasagiline and safinamide .
2. Antioxidant Activity
The antioxidant capacity of the compound was assessed using the Oxygen Radical Absorbance Capacity (ORAC) assay, yielding a result equivalent to 2.27 Trolox units. This suggests that the compound can scavenge free radicals effectively, which is beneficial in neuroprotective strategies .
3. Neuroprotective Effects
In vitro studies have shown that the compound possesses neuroprotective properties, likely due to its ability to inhibit oxidative stress and inflammation in neuronal cells. This aligns with findings that similar compounds with benzyloxy structures exhibit neuroprotective effects against oxidative damage .
Case Studies
Several case studies have been conducted to evaluate the pharmacological profiles of related compounds with similar structures:
- Case Study 1 : A derivative with a benzyloxy group was tested for its MAO-B inhibitory activity and showed promising results, reinforcing the hypothesis that modifications in the benzyloxy position can enhance bioactivity .
- Case Study 2 : A series of benzothiazole derivatives were synthesized and evaluated for their neuroprotective effects and antioxidant activities, demonstrating that structural modifications can lead to significant changes in biological activity .
Structure-Activity Relationship (SAR)
A detailed analysis of the structure-activity relationship indicates that:
- The presence of fluorine substituents enhances lipophilicity, potentially improving blood-brain barrier penetration.
- The benzyloxy group plays a crucial role in modulating enzyme inhibition and antioxidant activity.
Structural Feature | Effect on Activity |
---|---|
Fluorophenyl moiety | Increases lipophilicity and enzyme affinity |
Benzyloxy group | Enhances MAO-B inhibition and antioxidant capacity |
Oxopentanoate backbone | Contributes to overall stability and reactivity |
Properties
Molecular Formula |
C33H31F2NO4 |
---|---|
Molecular Weight |
543.6 g/mol |
IUPAC Name |
ethyl 2-[(S)-(4-fluoroanilino)-(4-phenylmethoxyphenyl)methyl]-5-(4-fluorophenyl)-5-oxopentanoate |
InChI |
InChI=1S/C33H31F2NO4/c1-2-39-33(38)30(20-21-31(37)24-8-12-26(34)13-9-24)32(36-28-16-14-27(35)15-17-28)25-10-18-29(19-11-25)40-22-23-6-4-3-5-7-23/h3-19,30,32,36H,2,20-22H2,1H3/t30?,32-/m1/s1 |
InChI Key |
OKCLDNDDYXKAMK-BDJZEIKTSA-N |
Isomeric SMILES |
CCOC(=O)C(CCC(=O)C1=CC=C(C=C1)F)[C@@H](C2=CC=C(C=C2)OCC3=CC=CC=C3)NC4=CC=C(C=C4)F |
Canonical SMILES |
CCOC(=O)C(CCC(=O)C1=CC=C(C=C1)F)C(C2=CC=C(C=C2)OCC3=CC=CC=C3)NC4=CC=C(C=C4)F |
Origin of Product |
United States |
Disclaimer and Information on In-Vitro Research Products
Please be aware that all articles and product information presented on BenchChem are intended solely for informational purposes. The products available for purchase on BenchChem are specifically designed for in-vitro studies, which are conducted outside of living organisms. In-vitro studies, derived from the Latin term "in glass," involve experiments performed in controlled laboratory settings using cells or tissues. It is important to note that these products are not categorized as medicines or drugs, and they have not received approval from the FDA for the prevention, treatment, or cure of any medical condition, ailment, or disease. We must emphasize that any form of bodily introduction of these products into humans or animals is strictly prohibited by law. It is essential to adhere to these guidelines to ensure compliance with legal and ethical standards in research and experimentation.