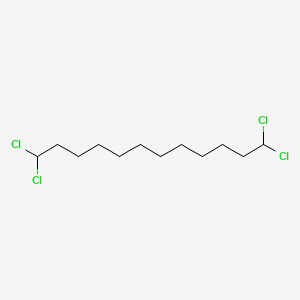
1,1,12,12-Tetrachlorododecane
- Click on QUICK INQUIRY to receive a quote from our team of experts.
- With the quality product at a COMPETITIVE price, you can focus more on your research.
Overview
Description
1,1,12,12-Tetrachlorododecane is an organic compound that belongs to the class of chlorinated hydrocarbons It is characterized by the presence of four chlorine atoms attached to a dodecane backbone
Preparation Methods
Synthetic Routes and Reaction Conditions
1,1,12,12-Tetrachlorododecane can be synthesized through the chlorination of dodecane. The process involves the reaction of dodecane with chlorine gas in the presence of a catalyst such as iron(III) chloride. The reaction is typically carried out under controlled conditions to ensure selective chlorination at the desired positions.
Industrial Production Methods
Industrial production of this compound follows similar principles but on a larger scale. The process involves continuous chlorination of dodecane in a reactor, with careful monitoring of temperature and chlorine concentration to optimize yield and purity. The product is then purified through distillation and other separation techniques.
Chemical Reactions Analysis
Types of Reactions
1,1,12,12-Tetrachlorododecane undergoes various chemical reactions, including:
Substitution Reactions: The chlorine atoms can be replaced by other functional groups through nucleophilic substitution reactions.
Oxidation Reactions: The compound can be oxidized to form corresponding alcohols or carboxylic acids.
Reduction Reactions: Reduction of this compound can lead to the formation of less chlorinated derivatives.
Common Reagents and Conditions
Substitution: Reagents such as sodium hydroxide or potassium hydroxide in an aqueous or alcoholic medium.
Oxidation: Oxidizing agents like potassium permanganate or chromium trioxide.
Reduction: Reducing agents such as lithium aluminum hydride or hydrogen gas in the presence of a catalyst.
Major Products
Substitution: Formation of alkylated derivatives.
Oxidation: Formation of dodecanol or dodecanoic acid.
Reduction: Formation of partially chlorinated dodecanes.
Scientific Research Applications
1,1,12,12-Tetrachlorododecane has several applications in scientific research:
Chemistry: Used as a reagent in organic synthesis and as a standard in analytical chemistry.
Biology: Studied for its potential effects on biological systems and its role as a model compound in toxicology studies.
Medicine: Investigated for its potential use in drug development and as a precursor for pharmacologically active compounds.
Industry: Utilized in the production of specialty chemicals and as an intermediate in the synthesis of other chlorinated compounds.
Mechanism of Action
The mechanism of action of 1,1,12,12-Tetrachlorododecane involves its interaction with cellular components. The compound can disrupt cell membranes and interfere with enzymatic processes due to its lipophilic nature. It may also form reactive intermediates that can bind to proteins and DNA, leading to potential toxic effects.
Comparison with Similar Compounds
Similar Compounds
- 1,2,9,10-Tetrachlorodecane
- 1,2,5,6,9,10-Hexachlorodecane
- 1,2,11,12-Tetrachlorododecane
Uniqueness
1,1,12,12-Tetrachlorododecane is unique due to its specific chlorination pattern, which imparts distinct chemical and physical properties. Compared to other similar compounds, it may exhibit different reactivity and biological activity, making it valuable for specific applications in research and industry.
Properties
Molecular Formula |
C12H22Cl4 |
---|---|
Molecular Weight |
308.1 g/mol |
IUPAC Name |
1,1,12,12-tetrachlorododecane |
InChI |
InChI=1S/C12H22Cl4/c13-11(14)9-7-5-3-1-2-4-6-8-10-12(15)16/h11-12H,1-10H2 |
InChI Key |
SJNWLDYYTNDOPD-UHFFFAOYSA-N |
Canonical SMILES |
C(CCCCCC(Cl)Cl)CCCCC(Cl)Cl |
Origin of Product |
United States |
Disclaimer and Information on In-Vitro Research Products
Please be aware that all articles and product information presented on BenchChem are intended solely for informational purposes. The products available for purchase on BenchChem are specifically designed for in-vitro studies, which are conducted outside of living organisms. In-vitro studies, derived from the Latin term "in glass," involve experiments performed in controlled laboratory settings using cells or tissues. It is important to note that these products are not categorized as medicines or drugs, and they have not received approval from the FDA for the prevention, treatment, or cure of any medical condition, ailment, or disease. We must emphasize that any form of bodily introduction of these products into humans or animals is strictly prohibited by law. It is essential to adhere to these guidelines to ensure compliance with legal and ethical standards in research and experimentation.