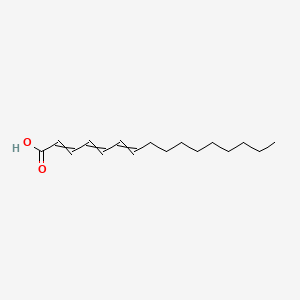
2,4,6-Hexadecatrienoic acid
- Click on QUICK INQUIRY to receive a quote from our team of experts.
- With the quality product at a COMPETITIVE price, you can focus more on your research.
Overview
Description
2,4,6-Hexadecatrienoic acid is a polyunsaturated fatty acid (PUFA) with the molecular formula C₁₆H₂₆O₂ (molecular weight: 250.38 g/mol) and three conjugated double bonds at positions 2, 4, and 6 in its all-trans (E,E,E) configuration . This structural arrangement distinguishes it from other hexadecatrienoic acid isomers, which typically feature non-conjugated or differently positioned double bonds. It has been identified in Dracocephalum palmatum and D. ruyschiana, plants used in traditional medicine, though its specific biological roles in these species remain understudied .
Preparation Methods
Synthetic Routes and Reaction Conditions
The synthesis of 2,4,6-Hexadecatrienoic acid typically involves the use of specific starting materials and reaction conditions to achieve the desired polyunsaturated structure. One common method involves the Wittig reaction, where a phosphonium ylide reacts with an aldehyde to form the desired triene structure. The reaction conditions often include the use of a strong base, such as sodium hydride, and an inert solvent like tetrahydrofuran .
Industrial Production Methods
Industrial production of this compound may involve the use of biotechnological approaches, such as the fermentation of genetically modified microorganisms capable of producing polyunsaturated fatty acids. These methods are advantageous due to their scalability and potential for sustainable production .
Chemical Reactions Analysis
Oxidation Reactions
2,4,6-Hexadecatrienoic acid undergoes oxidation through multiple pathways, primarily influenced by its three conjugated double bonds. Key reactions include:
Reaction Type | Reagents/Conditions | Products |
---|---|---|
Autoxidation | Oxygen (O₂), heat, light | Hydroperoxides, epoxides |
Enzymatic oxidation | Lipoxygenase (LOX), allene oxide synthase (AOS) | Dinor-oxo-phytodienoic acid (dn-OPDA) |
Chemical oxidation | Hydrogen peroxide (H₂O₂), catalytic metals (e.g., Fe²⁺) | Aldehydes, ketones, peroxides |
Autoxidation occurs spontaneously under ambient conditions, forming hydroperoxides and epoxides. Enzymatic pathways, such as those involving LOX and AOS in plants, convert the acid into dn-OPDA, a precursor to jasmonic acid (JA) in the hexadecanoid pathway .
Reduction Reactions
Reduction reactions primarily target the double bonds, yielding saturated fatty acids. Common methods include:
Reaction Type | Reagents/Conditions | Products |
---|---|---|
Catalytic hydrogenation | H₂, Pd/C, elevated pressure, temperature | Saturated hexadecanoic acid |
Chemical reduction | NaBH₄, LiAlH₄, acidic conditions | Partially saturated intermediates |
Catalytic hydrogenation is the most widely used method, requiring precise control of pressure and temperature to avoid over-reduction or side reactions .
Substitution Reactions
The carboxylic acid group enables esterification and amidation:
Esterification
Reaction Type | Reagents/Conditions | Products |
---|---|---|
Fischer esterification | Alcohols (e.g., methanol), HCl, H₂SO₄ | Methyl ester, ethyl ester, etc. |
Acid-catalyzed esterification | Acid catalysts (e.g., H₂SO₄), heat | Esters |
This reaction enhances solubility and stability, making derivatives suitable for industrial applications .
Amidation
Reaction Type | Reagents/Conditions | Products |
---|---|---|
Carbodiimide coupling | DCC, NHS, amines (e.g., glycine) | Amides |
Direct amidation | Amines, coupling agents (e.g., EDC) | N-acylated amines |
Amidation is critical for synthesizing bioactive compounds, such as lipid mediators involved in inflammatory responses .
Addition Reactions
The conjugated double bonds participate in electrophilic additions:
Reaction Type | Reagents/Conditions | Products |
---|---|---|
Hydrohalogenation | HX (e.g., HBr), light | Brominated/saturated derivatives |
Hydroboration | BH₃·THF, H₂O₂/NaOH | Alcohols |
Regioselectivity depends on the stability of intermediate carbocations. For example, hydrohalogenation favors addition at the terminal double bond .
Mechanistic Insights
The compound’s reactivity stems from its conjugated triene system and carboxylic acid group. Key mechanistic features:
-
Oxidative stability : Conjugated double bonds lower activation energy for radical formation.
-
Enzyme specificity : FAD5’s role in biosynthesis highlights structural dependence on Δ7-desaturation .
-
Substitution versatility : The carboxylic acid group enables diverse derivatization for tailored applications .
Scientific Research Applications
2,4,6-Hexadecatrienoic acid, also known as hexadeca-2,4,6-trienoic acid, is a long-chain polyunsaturated fatty acid with a 16-carbon backbone and three double bonds . It is a member of the long-chain fatty acids . This compound has roles in regulating inflammation, influencing cellular membrane fluidity, and potentially serving as a biomarker for dietary intake.
Chemical Reactions
9,12,15-Hexadecatrienoic acid can undergo several types of chemical reactions:
- Oxidation: This reaction can lead to the creation of hydroperoxides and other oxidation products. Common oxidizing agents include oxygen, ozone, and hydrogen peroxide.
- Reduction: Reduction reactions can convert double bonds into single bonds, resulting in a saturated fatty acid. Catalytic hydrogenation using hydrogen gas and a metal catalyst, such as palladium, is a typical method.
- Substitution: The carboxyl group of the fatty acid can participate in esterification and amidation reactions. Esterification can be carried out using alcohols and acid catalysts, while amidation involves amines and coupling agents.
Scientific Research Applications
9,12,15-Hexadecatrienoic acid has a wide range of applications in scientific research:
- Chemistry: It is used as a model compound to study the behavior of polyunsaturated fatty acids in various chemical reactions.
- Biology: The compound is involved in the study of lipid metabolism and the role of omega-3 fatty acids in cellular processes.
- Medicine: Research has shown its potential in anti-inflammatory and antibacterial applications.
- Industry: It is used in the production of bio-based materials and as a component in nutritional supplements.
Biological Activities
9,12,15-Hexadecatrienoic acid exhibits several biological activities:
- Anti-inflammatory and Antimicrobial Effects: Research indicates that this compound exhibits significant anti-inflammatory properties and can inhibit the growth of pathogenic bacteria such as Propionibacterium acnes. This suggests its potential application in dermatological treatments.
- Role in Lipid Metabolism: The compound acts as a precursor for bioactive lipid mediators involved in inflammation and immune responses. Its incorporation into cell membranes affects membrane fluidity and functionality, which is crucial for cellular signaling and metabolic processes.
- Anti-tumor Activity: Polysaccharides containing hexadecatrienoic acid have exhibited antitumor activity against MCF-7 breast cancer cells, suggesting a synergistic role in enhancing the overall bioactivity of the polysaccharides.
Mechanism of Action
The mechanism of action of 2,4,6-Hexadecatrienoic acid involves its incorporation into cell membranes, where it can influence membrane fluidity and function. It may also interact with specific molecular targets, such as enzymes involved in fatty acid metabolism. The pathways involved include the modulation of inflammatory responses and the regulation of gene expression related to lipid metabolism .
Comparison with Similar Compounds
Comparison with Structurally Similar Compounds
2,4,6-Hexadecatrienoic Acid
- Structure : Conjugated double bonds at C2, C4, and C6 (all-trans) .
- Sources : Isolated from Dracocephalum spp. .
- Biological Role: Not well-characterized, but hypothesized to participate in plant defense or signaling due to its presence in medicinal plants .
7,10,13-Hexadecatrienoic Acid
- Structure: Non-conjugated double bonds at C7, C10, and C13 (all-cis) .
- Sources : Found in Panax ginseng (anti-inflammatory glycosyl glycerides) and Lepidium sativum seeds (antimicrobial and antioxidant activity) .
- Biological Role : Contributes to anti-inflammatory and antimicrobial properties in plant extracts .
6,9,12-Hexadecatrienoic Acid
- Structure : Double bonds at C6, C9, and C12 (all-cis) .
- Sources : Major component of lipids in the marine crustacean Euphausia pacifica .
- Biological Role : Likely involved in membrane fluidity and energy storage in marine organisms .
9,12,15-Hexadecatrienoic Acid
- Structure : Double bonds at C9, C12, and C15 (E,E configuration) .
- Sources : Identified in spinach as a biomarker for dietary intake .
- Biological Role: Potential antioxidant activity, though less studied compared to other PUFAs .
Comparative Analysis of Properties and Functions
Metabolic and Functional Distinctions
- Oxylipin Pathways: 7,10,13-Hexadecatrienoic acid derivatives (e.g., 11-hydroperoxy hexadecatrienoic acid) are involved in wound response pathways in Physcomitrium patens .
- Enzymatic Specificity: Recombinant soybean lipoxygenase GmLOX2 converts hexadecatrienoic acid (likely 7,10,13 isomer) into 11-hydroperoxy and 7-hydroperoxy derivatives, indicating substrate specificity based on double bond positioning .
- Biosynthetic Routes: In Marchantia polymorpha, dn-OPDA (a jasmonate precursor) is synthesized from hexadecatrienoic acid, though the isomer involved is unspecified .
Analytical Differentiation
- GC-MS and LC-MS: Used to distinguish isomers via retention times and fragmentation patterns. For example, 7(Z),10(Z),13(Z)-hexadecatrienoic acid methyl ester was identified in Panax ginseng using GC-MS .
- Chiral Phase HPLC : Applied to determine stereochemistry of hydroperoxide derivatives (e.g., 11-S-HPHT from GmLOX2) .
Biological Activity
2,4,6-Hexadecatrienoic acid, also known as cis-6,9,12-hexadecatrienoic acid, is a polyunsaturated fatty acid with significant biological activity. Its structure features three double bonds, contributing to its unique properties and effects on various biological systems. This article aims to explore the biological activities of this compound, highlighting its roles in cellular processes, immunological responses, and potential therapeutic applications.
This compound has the molecular formula C₁₆H₂₆O₂ and a molecular weight of 254.39 g/mol. The presence of multiple double bonds allows it to participate in various biochemical reactions and interactions within biological membranes.
Structure
The structural formula of this compound can be represented as follows:
Membrane Fluidity and Flexibility
One of the primary biological activities of this compound is its role in regulating membrane fluidity and flexibility. This property is crucial for maintaining cellular integrity and function, particularly in neuronal tissues where fluidity affects signal transduction pathways .
Anti-inflammatory Effects
Research indicates that this compound exhibits anti-inflammatory properties. It modulates immune responses by influencing the production of pro-inflammatory cytokines and mediators. This fatty acid has been shown to interact with various signaling pathways involved in inflammation .
Antimicrobial Activity
This compound demonstrates antimicrobial properties against a range of pathogens. Studies have reported its effectiveness against bacteria and viruses, including HIV and influenza virus . The fatty acid's mechanism involves disrupting microbial membranes and inhibiting their growth.
Neuroprotective Effects
The compound has been associated with neuroprotective effects due to its ability to modulate neuronal signaling pathways. It influences neurotransmitter release and may play a role in preventing neurodegenerative diseases by reducing oxidative stress and inflammation in neural tissues .
Role in Metabolism
This compound is involved in lipid metabolism. It acts as a substrate for various metabolic enzymes and influences the synthesis of bioactive lipids that regulate metabolic processes such as insulin sensitivity and energy homeostasis .
Study on Inflammatory Response
A study published in the Journal of Biological Chemistry investigated the effects of this compound on macrophages. The results indicated that treatment with this fatty acid reduced the secretion of pro-inflammatory cytokines such as TNF-alpha and IL-6. This suggests a potential therapeutic role for this compound in managing inflammatory diseases .
Neuroprotective Study
Another research effort focused on the neuroprotective effects of this compound in a mouse model of Alzheimer's disease. The findings demonstrated that administration of this fatty acid improved cognitive function and reduced amyloid-beta accumulation in the brain. These results highlight its potential as a therapeutic agent for neurodegenerative conditions .
Data Table: Biological Activities of this compound
Biological Activity | Effect | Mechanism |
---|---|---|
Membrane Fluidity | Enhances cellular integrity | Regulates lipid bilayer dynamics |
Anti-inflammatory | Reduces cytokine production | Modulates immune signaling pathways |
Antimicrobial | Inhibits pathogen growth | Disrupts microbial membranes |
Neuroprotective | Improves cognitive function | Reduces oxidative stress |
Metabolic Regulation | Influences lipid metabolism | Serves as a substrate for metabolic enzymes |
Q & A
Q. Basic: What are the optimal methods for extracting and purifying 2,4,6-hexadecatrienoic acid from biological samples?
Methodological Answer :
The Bligh and Dyer method is widely used for lipid extraction due to its efficiency and reproducibility. Homogenize wet tissue with a chloroform-methanol mixture (2:1 v/v) to form a miscible system with tissue water. After dilution with chloroform and water, separate the chloroform layer containing lipids. Validate purity using HPLC-MS or GC-MS, ensuring solvent ratios are adjusted for polar lipids like trienoic acids . For targeted extraction, consider solid-phase extraction (SPE) with C18 cartridges to isolate unsaturated fatty acids, followed by thin-layer chromatography (TLC) for purity assessment .
Q. Basic: Which analytical techniques are most reliable for structural identification and quantification of this compound?
Methodological Answer :
Combine gas chromatography-mass spectrometry (GC-MS) with derivatization (e.g., methyl ester formation) to enhance volatility. For stereochemical confirmation, nuclear magnetic resonance (NMR) spectroscopy, particularly 13C-NMR, can resolve double-bond positions (e.g., 2,4,6 vs. 6,9,12 isomers). Quantify using high-resolution LC-MS with a reverse-phase C30 column to separate geometric isomers. Report detection limits and calibration curves using internal standards (e.g., deuterated fatty acids) .
Q. Advanced: How does this compound participate in mitochondrial β-oxidation pathways, and what experimental models are suitable for studying its metabolism?
Methodological Answer :
Design in vitro assays using liver or muscle mitochondria to assess β-oxidation rates. Prepare acyl-CoA derivatives of this compound and monitor NADH production via spectrophotometry. Compare activity with saturated analogs (e.g., palmitic acid) to evaluate enzyme specificity. Use knockout models (e.g., acyl-CoA dehydrogenase-deficient mice) to identify rate-limiting steps. Note that unsaturated positions (e.g., 2,4,6) may hinder dehydrogenation, requiring coupled assays with electron-transferring flavoprotein (ETF) .
Q. Advanced: How can researchers evaluate the antioxidant potential of this compound in cellular models, and what are common pitfalls?
Methodological Answer :
Use H2O2-induced oxidative stress in cell lines (e.g., HepG2 or SH-SY5Y) or pre-implantation embryos. Pre-treat cells with this compound (10–50 µM) and measure ROS levels via fluorescent probes (e.g., DCFH-DA). Assess mitochondrial membrane potential (MMP) using JC-1 staining. Include controls for solvent effects (e.g., dimethyl sulfoxide) and validate results with orthogonal methods (e.g., electron paramagnetic resonance). Pitfalls include isomer-specific effects; confirm double-bond positions to avoid conflating results with 6,9,12 isomers .
Q. Advanced: How should researchers address contradictions in published data on the bioactivity of this compound?
Methodological Answer :
Systematically evaluate sources of variability:
- Isomer purity : Verify via GC-MS/NMR, as commercial samples may contain mixed isomers .
- Model systems : Compare outcomes across in vitro (cell lines), ex vivo (isolated mitochondria), and in vivo (rodent) models.
- Dosage : Perform dose-response curves to identify non-linear effects.
- Replication : Use multi-lab collaborative studies to minimize technical bias. Meta-analyses of existing datasets can highlight trends obscured by small sample sizes .
Q. Advanced: What experimental design considerations are critical for studying this compound in developmental biology contexts?
Methodological Answer :
Adopt a blinded, randomized design for embryo culture studies. Use zygotes (mouse or donated human embryos) cultured in media supplemented with this compound (1–10 µM). Include oxidative stress controls (e.g., H2O2) and measure blastocyst formation rates. Ensure ethical compliance by obtaining informed consent for human embryos and adhering to ARRIVE guidelines for animal studies. Sample size calculations (e.g., power analysis) are essential to detect ≥20% improvement in development rates .
Q. Basic: What statistical approaches are recommended for analyzing dose-dependent effects of this compound?
Methodological Answer :
Apply ANOVA with post-hoc Tukey tests for multi-group comparisons. For non-linear relationships (e.g., hormetic effects), use sigmoidal curve fitting (Hill equation). Report effect sizes and confidence intervals. Include raw data in supplementary materials, and address outliers via Grubbs’ test or robust regression. For omics datasets, apply false discovery rate (FDR) correction .
Properties
Molecular Formula |
C16H26O2 |
---|---|
Molecular Weight |
250.38 g/mol |
IUPAC Name |
hexadeca-2,4,6-trienoic acid |
InChI |
InChI=1S/C16H26O2/c1-2-3-4-5-6-7-8-9-10-11-12-13-14-15-16(17)18/h10-15H,2-9H2,1H3,(H,17,18) |
InChI Key |
SZQQHKQCCBDXCG-UHFFFAOYSA-N |
Canonical SMILES |
CCCCCCCCCC=CC=CC=CC(=O)O |
Origin of Product |
United States |
Disclaimer and Information on In-Vitro Research Products
Please be aware that all articles and product information presented on BenchChem are intended solely for informational purposes. The products available for purchase on BenchChem are specifically designed for in-vitro studies, which are conducted outside of living organisms. In-vitro studies, derived from the Latin term "in glass," involve experiments performed in controlled laboratory settings using cells or tissues. It is important to note that these products are not categorized as medicines or drugs, and they have not received approval from the FDA for the prevention, treatment, or cure of any medical condition, ailment, or disease. We must emphasize that any form of bodily introduction of these products into humans or animals is strictly prohibited by law. It is essential to adhere to these guidelines to ensure compliance with legal and ethical standards in research and experimentation.