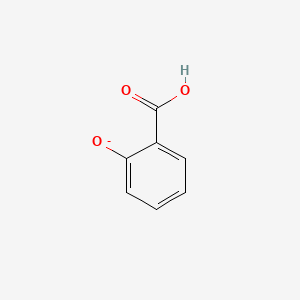
Salicylate
Overview
Description
Salicylates are a group of chemical compounds that are derivatives of salicylic acid. They are found naturally in various plants, such as willow bark and wintergreen leaves, and are known for their medicinal properties. Salicylic acid, the simplest form of salicylate, is widely used in pharmaceuticals, particularly in the production of aspirin (acetylsalicylic acid). Salicylates have anti-inflammatory, analgesic, and antipyretic properties, making them valuable in treating pain, fever, and inflammation .
Preparation Methods
Synthetic Routes and Reaction Conditions: Salicylic acid can be synthesized through several methods. One common method involves the Kolbe-Schmitt reaction, where sodium phenoxide reacts with carbon dioxide under high pressure and temperature to form sodium salicylate, which is then acidified to produce salicylic acid . Another method involves the hydrolysis of methyl this compound (oil of wintergreen) with sodium hydroxide, followed by acidification .
Industrial Production Methods: In industrial settings, salicylic acid is typically produced using the Kolbe-Schmitt reaction due to its efficiency and cost-effectiveness. The process involves the reaction of sodium phenoxide with carbon dioxide at temperatures around 125°C and pressures of 100 atmospheres. The resulting sodium this compound is then treated with sulfuric acid to yield salicylic acid .
Chemical Reactions Analysis
Types of Reactions: Salicylates undergo various chemical reactions, including:
Oxidation: Salicylic acid can be oxidized to produce catechol and other quinones.
Reduction: Reduction of salicylic acid can yield salicyl alcohol.
Substitution: Salicylic acid can undergo electrophilic aromatic substitution reactions, such as nitration, sulfonation, and halogenation.
Common Reagents and Conditions:
Oxidation: Potassium permanganate or chromic acid in acidic conditions.
Reduction: Lithium aluminum hydride or sodium borohydride in anhydrous conditions.
Major Products:
Oxidation: Catechol, quinones.
Reduction: Salicyl alcohol.
Substitution: Nitro-salicylic acid, sulfo-salicylic acid, halogenated salicylic acids.
Scientific Research Applications
Salicylates have a wide range of applications in scientific research:
Chemistry: Used as reagents in organic synthesis and as starting materials for the synthesis of various pharmaceuticals.
Biology: Studied for their role in plant defense mechanisms and as signaling molecules in plants.
Medicine: Widely used in the treatment of pain, inflammation, and fever.
Industry: Used in the production of dyes, preservatives, and as a flavoring agent in food and beverages.
Mechanism of Action
Salicylic acid exerts its effects primarily through the inhibition of cyclooxygenase (COX) enzymes, which are involved in the synthesis of prostaglandins. By inhibiting COX-1 and COX-2, salicylic acid reduces the formation of pro-inflammatory prostaglandins, leading to its anti-inflammatory and analgesic effects. Additionally, salicylic acid has keratolytic properties, making it effective in treating skin conditions by promoting the shedding of the outer layer of skin .
Comparison with Similar Compounds
Salicylates are part of a larger group of compounds known as nonsteroidal anti-inflammatory drugs (NSAIDs). Similar compounds include:
Aspirin (Acetylsalicylic Acid): A derivative of salicylic acid with acetylation at the hydroxyl group, providing enhanced anti-inflammatory and analgesic properties.
Methyl Salicylate: An ester of salicylic acid used as a flavoring agent and in topical pain relief products.
Diflunisal: A salicylic acid derivative with a difluorophenyl group, offering longer-lasting analgesic effects.
Magnesium this compound: A salt of salicylic acid used as an analgesic and anti-inflammatory agent.
Uniqueness: Salicylic acid is unique due to its dual role as a precursor to aspirin and its direct use in topical treatments for skin conditions. Its ability to inhibit COX enzymes and promote keratolysis distinguishes it from other salicylates .
Properties
IUPAC Name |
2-carboxyphenolate | |
---|---|---|
Details | Computed by Lexichem TK 2.7.0 (PubChem release 2021.10.14) | |
Source | PubChem | |
URL | https://pubchem.ncbi.nlm.nih.gov | |
Description | Data deposited in or computed by PubChem | |
InChI |
InChI=1S/C7H6O3/c8-6-4-2-1-3-5(6)7(9)10/h1-4,8H,(H,9,10)/p-1 | |
Details | Computed by InChI 1.0.6 (PubChem release 2021.10.14) | |
Source | PubChem | |
URL | https://pubchem.ncbi.nlm.nih.gov | |
Description | Data deposited in or computed by PubChem | |
InChI Key |
YGSDEFSMJLZEOE-UHFFFAOYSA-M | |
Details | Computed by InChI 1.0.6 (PubChem release 2021.10.14) | |
Source | PubChem | |
URL | https://pubchem.ncbi.nlm.nih.gov | |
Description | Data deposited in or computed by PubChem | |
Canonical SMILES |
C1=CC=C(C(=C1)C(=O)O)[O-] | |
Details | Computed by OEChem 2.3.0 (PubChem release 2021.10.14) | |
Source | PubChem | |
URL | https://pubchem.ncbi.nlm.nih.gov | |
Description | Data deposited in or computed by PubChem | |
Molecular Formula |
C7H5O3- | |
Details | Computed by PubChem 2.2 (PubChem release 2021.10.14) | |
Source | PubChem | |
URL | https://pubchem.ncbi.nlm.nih.gov | |
Description | Data deposited in or computed by PubChem | |
DSSTOX Substance ID |
DTXSID70948389 | |
Record name | 2-Carboxyphenolate | |
Source | EPA DSSTox | |
URL | https://comptox.epa.gov/dashboard/DTXSID70948389 | |
Description | DSSTox provides a high quality public chemistry resource for supporting improved predictive toxicology. | |
Molecular Weight |
137.11 g/mol | |
Details | Computed by PubChem 2.2 (PubChem release 2021.10.14) | |
Source | PubChem | |
URL | https://pubchem.ncbi.nlm.nih.gov | |
Description | Data deposited in or computed by PubChem | |
CAS No. |
63-36-5, 25496-36-0 | |
Record name | Salicylate | |
Source | CAS Common Chemistry | |
URL | https://commonchemistry.cas.org/detail?cas_rn=63-36-5 | |
Description | CAS Common Chemistry is an open community resource for accessing chemical information. Nearly 500,000 chemical substances from CAS REGISTRY cover areas of community interest, including common and frequently regulated chemicals, and those relevant to high school and undergraduate chemistry classes. This chemical information, curated by our expert scientists, is provided in alignment with our mission as a division of the American Chemical Society. | |
Explanation | The data from CAS Common Chemistry is provided under a CC-BY-NC 4.0 license, unless otherwise stated. | |
Record name | Salicylate | |
Source | ChemIDplus | |
URL | https://pubchem.ncbi.nlm.nih.gov/substance/?source=chemidplus&sourceid=0000063365 | |
Description | ChemIDplus is a free, web search system that provides access to the structure and nomenclature authority files used for the identification of chemical substances cited in National Library of Medicine (NLM) databases, including the TOXNET system. | |
Record name | 2-Carboxyphenolate | |
Source | EPA DSSTox | |
URL | https://comptox.epa.gov/dashboard/DTXSID70948389 | |
Description | DSSTox provides a high quality public chemistry resource for supporting improved predictive toxicology. | |
Retrosynthesis Analysis
AI-Powered Synthesis Planning: Our tool employs the Template_relevance Pistachio, Template_relevance Bkms_metabolic, Template_relevance Pistachio_ringbreaker, Template_relevance Reaxys, Template_relevance Reaxys_biocatalysis model, leveraging a vast database of chemical reactions to predict feasible synthetic routes.
One-Step Synthesis Focus: Specifically designed for one-step synthesis, it provides concise and direct routes for your target compounds, streamlining the synthesis process.
Accurate Predictions: Utilizing the extensive PISTACHIO, BKMS_METABOLIC, PISTACHIO_RINGBREAKER, REAXYS, REAXYS_BIOCATALYSIS database, our tool offers high-accuracy predictions, reflecting the latest in chemical research and data.
Strategy Settings
Precursor scoring | Relevance Heuristic |
---|---|
Min. plausibility | 0.01 |
Model | Template_relevance |
Template Set | Pistachio/Bkms_metabolic/Pistachio_ringbreaker/Reaxys/Reaxys_biocatalysis |
Top-N result to add to graph | 6 |
Feasible Synthetic Routes
Disclaimer and Information on In-Vitro Research Products
Please be aware that all articles and product information presented on BenchChem are intended solely for informational purposes. The products available for purchase on BenchChem are specifically designed for in-vitro studies, which are conducted outside of living organisms. In-vitro studies, derived from the Latin term "in glass," involve experiments performed in controlled laboratory settings using cells or tissues. It is important to note that these products are not categorized as medicines or drugs, and they have not received approval from the FDA for the prevention, treatment, or cure of any medical condition, ailment, or disease. We must emphasize that any form of bodily introduction of these products into humans or animals is strictly prohibited by law. It is essential to adhere to these guidelines to ensure compliance with legal and ethical standards in research and experimentation.