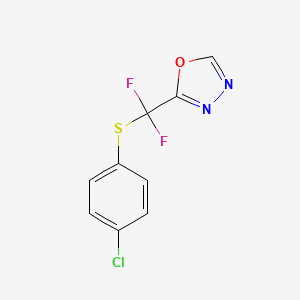
2-(((4-Chlorophenyl)thio)difluoromethyl)-1,3,4-oxadiazole
- Click on QUICK INQUIRY to receive a quote from our team of experts.
- With the quality product at a COMPETITIVE price, you can focus more on your research.
Overview
Description
2-(((4-Chlorophenyl)thio)difluoromethyl)-1,3,4-oxadiazole is a heterocyclic compound that contains an oxadiazole ring substituted with a difluoromethyl group and a 4-chlorophenylthio group
Preparation Methods
Synthetic Routes and Reaction Conditions
The synthesis of 2-(((4-Chlorophenyl)thio)difluoromethyl)-1,3,4-oxadiazole typically involves the reaction of 4-chlorothiophenol with difluoromethylating agents followed by cyclization with appropriate reagents to form the oxadiazole ring. One common method involves the use of sodium hydride as a base and dimethylformamide as a solvent under reflux conditions .
Industrial Production Methods
Industrial production of this compound may involve similar synthetic routes but optimized for large-scale production. This includes the use of continuous flow reactors and automated systems to ensure consistent quality and yield. The reaction conditions are carefully controlled to minimize by-products and maximize the efficiency of the process.
Chemical Reactions Analysis
Types of Reactions
2-(((4-Chlorophenyl)thio)difluoromethyl)-1,3,4-oxadiazole can undergo various chemical reactions, including:
Oxidation: The compound can be oxidized using strong oxidizing agents such as potassium permanganate or hydrogen peroxide.
Reduction: Reduction reactions can be carried out using reducing agents like lithium aluminum hydride.
Substitution: The compound can undergo nucleophilic substitution reactions, particularly at the 4-chlorophenylthio group.
Common Reagents and Conditions
Oxidation: Potassium permanganate in acidic medium.
Reduction: Lithium aluminum hydride in anhydrous ether.
Substitution: Sodium hydride in dimethylformamide.
Major Products Formed
Oxidation: Formation of sulfoxides or sulfones.
Reduction: Formation of thiols or thioethers.
Substitution: Formation of various substituted oxadiazoles.
Scientific Research Applications
Chemistry: Used as a building block for the synthesis of more complex molecules.
Biology: Investigated for its antimicrobial and antifungal properties.
Medicine: Potential use as a pharmaceutical intermediate for the development of new drugs.
Industry: Utilized in the development of agrochemicals and materials with specific properties.
Mechanism of Action
The mechanism of action of 2-(((4-Chlorophenyl)thio)difluoromethyl)-1,3,4-oxadiazole involves its interaction with specific molecular targets. In biological systems, it may inhibit the activity of certain enzymes or disrupt cellular processes by binding to specific receptors or proteins. The exact pathways and molecular targets can vary depending on the specific application and the biological system being studied .
Comparison with Similar Compounds
Similar Compounds
- 2-(((4-Bromophenyl)thio)difluoromethyl)-1,3,4-oxadiazole
- 2-(((4-Methylphenyl)thio)difluoromethyl)-1,3,4-oxadiazole
- 2-(((4-Fluorophenyl)thio)difluoromethyl)-1,3,4-oxadiazole
Uniqueness
2-(((4-Chlorophenyl)thio)difluoromethyl)-1,3,4-oxadiazole is unique due to the presence of the 4-chlorophenylthio group, which imparts specific chemical and biological properties.
Properties
Molecular Formula |
C9H5ClF2N2OS |
---|---|
Molecular Weight |
262.66 g/mol |
IUPAC Name |
2-[(4-chlorophenyl)sulfanyl-difluoromethyl]-1,3,4-oxadiazole |
InChI |
InChI=1S/C9H5ClF2N2OS/c10-6-1-3-7(4-2-6)16-9(11,12)8-14-13-5-15-8/h1-5H |
InChI Key |
ZLGAIATWLOAHJE-UHFFFAOYSA-N |
Canonical SMILES |
C1=CC(=CC=C1SC(C2=NN=CO2)(F)F)Cl |
Origin of Product |
United States |
Disclaimer and Information on In-Vitro Research Products
Please be aware that all articles and product information presented on BenchChem are intended solely for informational purposes. The products available for purchase on BenchChem are specifically designed for in-vitro studies, which are conducted outside of living organisms. In-vitro studies, derived from the Latin term "in glass," involve experiments performed in controlled laboratory settings using cells or tissues. It is important to note that these products are not categorized as medicines or drugs, and they have not received approval from the FDA for the prevention, treatment, or cure of any medical condition, ailment, or disease. We must emphasize that any form of bodily introduction of these products into humans or animals is strictly prohibited by law. It is essential to adhere to these guidelines to ensure compliance with legal and ethical standards in research and experimentation.