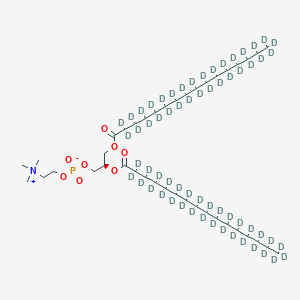
1,2-Dipalmitoyl-d62-sn-glycero-3-phosphocholine
Overview
Description
1,2-Dipalmitoyl-d62-sn-glycero-3-phosphocholine (DPPC-d62) is a deuterated analog of the saturated phospholipid DPPC, where all hydrogens in the palmitoyl chains (C16:0) are replaced with deuterium (d62). This isotopic substitution retains the chemical structure of the parent molecule but introduces distinct physicochemical properties, particularly in spectroscopic and neutron scattering studies . DPPC-d62 is widely used in membrane biophysics to investigate lipid bilayer phase behavior, conformational order, and interactions with ions or proteins . Its phase transition temperature (~41°C for non-deuterated DPPC) is slightly altered due to isotopic effects, making it valuable for probing gel-to-liquid crystalline transitions .
Preparation Methods
Synthetic Routes and Reaction Conditions: 1,2-Dipalmitoyl-d62-sn-glycero-3-phosphocholine can be synthesized through the esterification of palmitic acid with deuterated glycerol phosphocholine. The reaction typically involves the use of a catalyst and is carried out under controlled temperature and pressure conditions to ensure the complete substitution of hydrogen with deuterium .
Industrial Production Methods: In industrial settings, the production of this compound involves large-scale esterification processes. The use of high-purity reagents and advanced purification techniques ensures the compound’s high deuterium content and purity .
Chemical Reactions Analysis
Esterification and Acyl Chain Modification
DPPC-d62 undergoes regioselective esterification for controlled chain substitution. In enzyme-assisted synthesis:
-
sn-2 position esterification : Achieved using oleic anhydride-d64 and DMAP catalyst in dichloromethane (DCM), yielding 1-palmitoyl-d31-2-oleoyl-d32-sn-glycero-3-phosphocholine (POPC-d63) with 72% efficiency .
-
sn-1 position substitution : Enzymatic hydrolysis with Rhizomucor miehei lipase (RM lipase) at 40°C in ethanol removes the native palmitoyl chain. Subsequent esterification with deuterated palmitic acid-d31 produces regiopure DPPC-d62 with >96% chemical purity .
Key parameters :
Reaction Step | Catalyst/Conditions | Yield (%) | Purity (%) |
---|---|---|---|
sn-2 esterification | DMAP, DCM, RT | 72 | 89 |
sn-1 substitution | RM lipase, 40°C | 85 | 96.4 |
Acyl Migration and Side Reactions
Acyl migration introduces regioisomers during synthesis:
-
Mechanism : Acid/base-catalyzed migration of acyl groups between sn-1 and sn-2 positions occurs in organic solvents (e.g., chloroform), forming 1-oleoyl-2-palmitoyl-sn-glycero-3-phosphocholine (OPPC-d63) .
-
Mitigation : Enzymatic specificity (sn-1,3-specific RM lipase) suppresses regioisomer formation, limiting OPPC-d63 contamination to <3.6% .
Isotopologue distribution :
Component | Proportion (%) |
---|---|
POPC-d63 | 96.4 |
DPPC-d31/d62 | 3.6 |
Transesterification for Analytical Characterization
DPPC-d62 is transesterified to fatty acid methyl esters (FAMEs) for chain analysis:
-
Method : Reaction with anhydrous methanolic HCl converts deuterated acyl chains to methyl palmitate-d31 and methyl oleate-d32.
-
Quantification : GC-FID confirms chain ratios using relative molar response (RMR) factors, unaffected by deuterium substitution .
Phase Behavior and Conformational Dynamics
While primarily physical, DPPC-d62’s phase transitions influence reaction kinetics:
-
Liquid-ordered (Lo) phase : In DOPC/DPPC-d62/cholesterol mixtures, DPPC-d62 adopts ordered all-trans conformations below 25°C, impacting reactivity in bilayer environments .
-
Activation energy : Lipid flip-flop in bilayers requires 220 kJ/mol, measured via sum-frequency vibrational spectroscopy (SFVS) .
Stability Under Oxidative and Thermal Stress
-
Oxidation : Susceptible to radical-mediated oxidation at unsaturated sites (e.g., introduced oleoyl chains), forming hydroperoxides .
-
Thermal degradation : Decomposes above 229°C, with α-D23 optical rotation = +6.6° (c = 4.2 in chloroform/methanol) .
Synthetic Byproducts and Purification
Scientific Research Applications
Lipid Bilayer and Membrane Studies
DPPC-d62 is primarily utilized in the study of lipid bilayers and membrane dynamics due to its ability to form stable lipid structures. It serves as a model compound for understanding the properties of biological membranes.
- Formation of Liposomes : DPPC-d62 is used to create liposomes, which are spherical vesicles that can encapsulate drugs or other compounds for targeted delivery. This application is crucial in drug delivery systems and vaccine development.
- Phase Transition Analysis : Studies have shown that DPPC undergoes phase transitions that are essential for understanding membrane fluidity and permeability. For instance, Raman spectroscopy has been employed to analyze the phase behavior of DPPC liposomes under various conditions, revealing insights into their structural properties .
Drug Delivery Systems
DPPC-d62 plays a significant role in enhancing the efficacy of drug delivery systems:
- Encapsulation Efficiency : Research indicates that liposomes made from DPPC can improve the encapsulation efficiency of hydrophobic drugs, such as curcumin, by providing a stable environment that enhances their solubility and bioavailability .
- Targeted Delivery : The incorporation of specific ligands into DPPC-based liposomes can facilitate targeted delivery to specific cell types, enhancing therapeutic outcomes in cancer treatment and other diseases .
NMR and Spectroscopy Applications
Due to its deuterated nature, DPPC-d62 is particularly useful in nuclear magnetic resonance (NMR) spectroscopy:
- Structural Analysis : The deuterium labeling allows for clearer spectral analysis, enabling researchers to study the dynamics and conformational changes of lipid molecules within membranes .
- Interaction Studies : NMR studies utilizing DPPC-d62 can provide insights into how drugs interact with lipid membranes, which is vital for developing new pharmaceuticals .
Immunological Applications
DPPC-d62 has been explored in immunological studies:
- Vaccine Development : Incorporation of glycosphingolipid antigens into DPPC-containing liposomes has been shown to enhance immunogenicity in animal models, suggesting potential applications in vaccine formulation .
Biophysical Research
The compound is also significant in biophysical research:
- Model Membranes : DPPC-d62 is used to create model membranes that simulate biological systems. This allows researchers to study membrane properties, such as fluidity and permeability, under controlled conditions .
- Protein Interactions : Studies have demonstrated that the composition of lipid membranes, including those made with DPPC-d62, affects protein adsorption behavior. This is crucial for understanding membrane protein functions .
Table 1: Summary of Applications
Mechanism of Action
1,2-Dipalmitoyl-d62-sn-glycero-3-phosphocholine exerts its effects by integrating into lipid bilayers, thereby altering membrane fluidity and stability. It interacts with membrane proteins and other lipids, influencing various cellular processes. The deuterium atoms enhance the compound’s stability, making it a valuable tool in studying membrane dynamics .
Comparison with Similar Compounds
Non-Deuterated DPPC
- Structure : Both DPPC and DPPC-d62 share identical headgroups (phosphocholine) and acyl chains (C16:0), but DPPC-d62’s deuterated chains enhance neutron scattering contrast and Raman spectral resolution .
- Applications: DPPC-d62 is critical in studies requiring isotopic labeling, such as neutron reflectometry (SNR) and dynamic nuclear polarization (DNP), whereas non-deuterated DPPC is used in calorimetry and conventional microscopy .
Phosphatidylcholines with Varying Acyl Chains
Key Differences :
- Chain Length : Longer chains (e.g., DSPC) increase bilayer thickness and transition temperatures, while shorter chains (e.g., DMPC) enhance membrane fluidity .
- Saturation : Unsaturated lipids like DOPC introduce kinks, reducing packing density and phase transition temperatures compared to saturated DPPC-d62 .
Phospholipids with Different Headgroups
- DPPS (Phosphatidylserine) : Negatively charged headgroup promotes calcium/magnium binding, influencing membrane fusion and protein recruitment . Unlike zwitterionic DPPC-d62, DPPS is used to study charge-dependent interactions .
- DPPE (Phosphatidylethanolamine): Smaller headgroup increases curvature stress, favoring non-lamellar phases in mixtures with DPPC-d62 .
Deuterated Analogues
- POPC-d31 : Partially deuterated (one chain) for tracking specific lipid regions in mixed membranes, unlike fully deuterated DPPC-d62 .
- d62-diC16:0 vs. diC22:0PC : DPPC-d62 (C16:0) forms thinner bilayers than diC22:0PC (C22:0), impacting membrane protein insertion and solvent accessibility .
Spectroscopic Advantages of DPPC-d62
- Raman Spectroscopy : The C-D stretching band (~2070–2300 cm⁻¹) in DPPC-d62 avoids overlap with C-H signals, enabling precise tracking of lipid order in mixed systems .
- Neutron Reflectometry : DPPC-d62’s distinct neutron scattering length density (SLD) allows resolution of bilayer substructures, such as headgroup and acyl chain regions .
Phase Behavior and Mechanical Properties
- DPPC-d62 bilayers exhibit reduced plasticity in stacked configurations due to hydration layers between headgroups, as revealed by nanomechanical mapping .
- In mixtures with cholesterol, DPPC-d62’s deuterated chains enable independent analysis of lipid conformational states via Raman spectroscopy .
Practical Considerations
- Cost: Deuterated lipids like DPPC-d62 are significantly more expensive than non-deuterated analogs (e.g., ~€234/g vs. ~€53–86/g for DPPC) .
- Sensitivity : Deuteration enhances detection limits in imaging but requires specialized handling to avoid hydrogen-deuterium exchange .
Biological Activity
1,2-Dipalmitoyl-d62-sn-glycero-3-phosphocholine (DPPC-d62) is a deuterated phospholipid widely used in biological and biochemical research. This compound is notable for its role in studying lipid membranes due to its unique properties and stability. The deuteration enhances its NMR spectroscopic characteristics, making it a valuable tool in membrane studies.
Chemical Structure and Properties
DPPC-d62 is a synthetic phospholipid where hydrogen atoms in the fatty acid chains are replaced with deuterium. This alteration influences its physical and chemical behaviors, particularly in membrane dynamics.
- Chemical Formula : CHDNOP
- Molecular Weight : Approximately 420.6 g/mol
- CAS Number : 25582-63-2
DPPC-d62 integrates into lipid bilayers, where it influences membrane fluidity and permeability. Its amphipathic nature allows it to interact with both hydrophilic and hydrophobic environments, affecting various biochemical pathways including:
- Phospholipid Metabolism : As a component of cell membranes, it plays a critical role in the metabolism of other lipids.
- Signal Transduction : Modulates the function of membrane proteins, impacting cellular signaling processes.
Pharmacokinetics
The pharmacokinetics of DPPC-d62 involve absorption through the intestine, incorporation into chylomicrons, and subsequent transport via the lymphatic system into the bloodstream. It can be taken up by cells and integrated into their membranes, influencing cellular functions.
Membrane Dynamics
Research indicates that DPPC-d62 significantly affects membrane properties. Studies employing NMR spectroscopy have demonstrated its role in liquid domain formation within lipid bilayers. For instance, the presence of cholesterol in DPPC-d62 membranes alters phase behavior and stability, leading to distinct liquid-ordered and liquid-disordered phases .
Case Studies
- Phase Transition Behavior : A study explored the impact of 2,4-dichlorophenol on DPPC liposomes using FT-Raman spectroscopy. Results showed that DCP alters the phase transition temperatures and structural integrity of DPPC membranes, indicating that environmental factors can significantly influence membrane dynamics .
- Drug Delivery Systems : Research involving glycerol's interaction with DPPC-d62 highlighted its potential to modify drug transport across cellular membranes. Glycerol increased the size of the phospholipid headgroup solvation shell and reduced bilayer thickness, which could enhance or restrict drug permeability depending on the formulation .
- NMR Studies : A study utilizing DPPC-d62 in planar-supported lipid bilayers measured flip-flop rates of lipids within membranes. Results indicated that temperature and lipid composition significantly impacted translocation rates, providing insights into lipid dynamics under physiological conditions .
Summary of Findings
Q & A
Q. Basic Synthesis and Characterization
Q. Q: What are the established methods for synthesizing and purifying deuterated DPPC (DPPC-d62)?
A: DPPC-d62 is synthesized via acylation of glycerophosphorylcholine (GPC) with deuterated palmitic acid anhydrides. Purification typically involves crystallization for saturated analogs (e.g., DPPC-d62) and column chromatography for unsaturated variants. For example, 1,2-dipalmitoyl-sn-3-GPC analogs are crystallized from acetone at low temperatures (−7°C) to achieve high purity (>99%) . Key challenges include minimizing isotopic scrambling during acylation and ensuring deuteration efficiency in the acyl chains.
Q. Advanced Analytical Applications
Q. Q: How does deuteration of DPPC enhance its utility in Raman spectroscopy and lipid bilayer studies?
A: Deuteration shifts C-H vibrational modes (~2800–3100 cm⁻¹) to distinct C-D signals (~2000–2300 cm⁻¹), enabling independent tracking of deuterated and non-deuterated lipids in mixed systems. This is critical for studying phase separation, conformational order, and lipid packing in model membranes. For instance, DPPC-d62 in DOPC/Chol mixtures allows isolation of chain-ordering effects in Raman spectra . Additionally, C-D bonds improve sensitivity in imaging lipid nanocarriers by reducing spectral overlap with biological matrices .
Q. Experimental Design for Membrane Studies
Q. Q: What protocols are recommended for preparing lipid vesicles containing DPPC-d62 for structural studies (e.g., SAXS, NMR)?
A:
- Thin-film hydration : Dissolve DPPC-d62 in chloroform, evaporate to form a film, and hydrate with buffer (e.g., ultra-pure water) above the lipid’s phase transition temperature (~41°C for DPPC). Vortexing or extrusion produces unilamellar vesicles .
- Deuteration compatibility : Ensure deuterated lipids are mixed with non-deuterated components at controlled ratios (e.g., 1:1 molar for phase studies) to avoid isotopic dilution effects .
- Validation : Confirm vesicle size via dynamic light scattering (DLS) and lamellar spacing via SAXS .
Q. Resolving Data Contradictions in Phase Behavior
Q. Q: How can discrepancies in lipid phase behavior observed via ESR and other techniques be reconciled?
A: ESR studies of DPPC-d62 in low-hydration bilayers reveal long-range cooperative chain distortions (e.g., bend/kink propagation) that may not align with DSC or SAXS data. To resolve contradictions:
- Cross-validate methods : Combine ESR with ²H NMR to assess both chain dynamics (ESR) and orientational order (NMR) .
- Control hydration : Discrepancies often arise from hydration differences; maintain consistent water content (2–15 wt%) for oriented multilayer studies .
- Probe selection : Use headgroup-specific spin labels (e.g., PD-Tempone) to isolate region-specific effects .
Q. Investigating Cation-Lipid Interactions
Q. Q: How do divalent cations like Mg²⁺ influence DPPC-d62 membrane organization?
A: Mg²⁺ induces microdomain reorganization in DPPC-d62/DPPS mixtures by neutralizing negative charges on PS headgroups, promoting phase separation. Key steps:
- Sample preparation : Incorporate 1–10 mM MgCl₂ into lipid suspensions.
- Detection : Use fluorescence quenching (e.g., with Laurdan) or deuterium NMR to track changes in lipid packing and phase boundaries .
- Thermodynamic analysis : Monitor shifts in main phase transition temperature (Tₘ) via DSC; Mg²⁺ typically increases Tₘ by ~2–3°C in mixed systems .
Q. Isotopic Purity and Storage
Q. Q: What quality control measures ensure isotopic integrity and stability of DPPC-d62?
A:
- Purity validation : Confirm >98% deuteration via mass spectrometry or ²H NMR. Commercial batches (e.g., Avanti Polar Lipids) often provide certificates of analysis .
- Storage : Store lyophilized DPPC-d62 at −20°C under argon to prevent oxidation. Reconstitute in deuterated solvents (e.g., D₂O) for NMR studies .
- Stability testing : Regularly assess lipid integrity using TLC or MALDI-TOF to detect hydrolytic or oxidative degradation .
Q. Advanced Applications in Drug Delivery
Q. Q: How does DPPC-d62 improve the specificity of intracellular nanocarrier imaging?
A: Deuteration enhances Raman signal-to-noise ratios in biological environments. For example:
- Cationic liposomes : DPPC-d62 tagged with DOTAP or SA shows distinct C-D peaks (~2070–2300 cm⁻¹), enabling tracking of intracellular distribution without interference from endogenous lipids .
- Quantitative imaging : Use spectral unmixing algorithms to differentiate deuterated carriers from background signals in live cells .
Properties
IUPAC Name |
[(2R)-2,3-bis(2,2,3,3,4,4,5,5,6,6,7,7,8,8,9,9,10,10,11,11,12,12,13,13,14,14,15,15,16,16,16-hentriacontadeuteriohexadecanoyloxy)propyl] 2-(trimethylazaniumyl)ethyl phosphate | |
---|---|---|
Source | PubChem | |
URL | https://pubchem.ncbi.nlm.nih.gov | |
Description | Data deposited in or computed by PubChem | |
InChI |
InChI=1S/C40H80NO8P/c1-6-8-10-12-14-16-18-20-22-24-26-28-30-32-39(42)46-36-38(37-48-50(44,45)47-35-34-41(3,4)5)49-40(43)33-31-29-27-25-23-21-19-17-15-13-11-9-7-2/h38H,6-37H2,1-5H3/t38-/m1/s1/i1D3,2D3,6D2,7D2,8D2,9D2,10D2,11D2,12D2,13D2,14D2,15D2,16D2,17D2,18D2,19D2,20D2,21D2,22D2,23D2,24D2,25D2,26D2,27D2,28D2,29D2,30D2,31D2,32D2,33D2 | |
Source | PubChem | |
URL | https://pubchem.ncbi.nlm.nih.gov | |
Description | Data deposited in or computed by PubChem | |
InChI Key |
KILNVBDSWZSGLL-PWXLRKPBSA-N | |
Source | PubChem | |
URL | https://pubchem.ncbi.nlm.nih.gov | |
Description | Data deposited in or computed by PubChem | |
Canonical SMILES |
CCCCCCCCCCCCCCCC(=O)OCC(COP(=O)([O-])OCC[N+](C)(C)C)OC(=O)CCCCCCCCCCCCCCC | |
Source | PubChem | |
URL | https://pubchem.ncbi.nlm.nih.gov | |
Description | Data deposited in or computed by PubChem | |
Isomeric SMILES |
[2H]C([2H])([2H])C([2H])([2H])C([2H])([2H])C([2H])([2H])C([2H])([2H])C([2H])([2H])C([2H])([2H])C([2H])([2H])C([2H])([2H])C([2H])([2H])C([2H])([2H])C([2H])([2H])C([2H])([2H])C([2H])([2H])C([2H])([2H])C(=O)OC[C@H](COP(=O)([O-])OCC[N+](C)(C)C)OC(=O)C([2H])([2H])C([2H])([2H])C([2H])([2H])C([2H])([2H])C([2H])([2H])C([2H])([2H])C([2H])([2H])C([2H])([2H])C([2H])([2H])C([2H])([2H])C([2H])([2H])C([2H])([2H])C([2H])([2H])C([2H])([2H])C([2H])([2H])[2H] | |
Source | PubChem | |
URL | https://pubchem.ncbi.nlm.nih.gov | |
Description | Data deposited in or computed by PubChem | |
Molecular Formula |
C40H80NO8P | |
Source | PubChem | |
URL | https://pubchem.ncbi.nlm.nih.gov | |
Description | Data deposited in or computed by PubChem | |
DSSTOX Substance ID |
DTXSID40677033 | |
Record name | (2R)-2,3-Bis[(~2~H_31_)hexadecanoyloxy]propyl 2-(trimethylazaniumyl)ethyl phosphate | |
Source | EPA DSSTox | |
URL | https://comptox.epa.gov/dashboard/DTXSID40677033 | |
Description | DSSTox provides a high quality public chemistry resource for supporting improved predictive toxicology. | |
Molecular Weight |
796.4 g/mol | |
Source | PubChem | |
URL | https://pubchem.ncbi.nlm.nih.gov | |
Description | Data deposited in or computed by PubChem | |
CAS No. |
25582-63-2 | |
Record name | (2R)-2,3-Bis[(~2~H_31_)hexadecanoyloxy]propyl 2-(trimethylazaniumyl)ethyl phosphate | |
Source | EPA DSSTox | |
URL | https://comptox.epa.gov/dashboard/DTXSID40677033 | |
Description | DSSTox provides a high quality public chemistry resource for supporting improved predictive toxicology. | |
Retrosynthesis Analysis
AI-Powered Synthesis Planning: Our tool employs the Template_relevance Pistachio, Template_relevance Bkms_metabolic, Template_relevance Pistachio_ringbreaker, Template_relevance Reaxys, Template_relevance Reaxys_biocatalysis model, leveraging a vast database of chemical reactions to predict feasible synthetic routes.
One-Step Synthesis Focus: Specifically designed for one-step synthesis, it provides concise and direct routes for your target compounds, streamlining the synthesis process.
Accurate Predictions: Utilizing the extensive PISTACHIO, BKMS_METABOLIC, PISTACHIO_RINGBREAKER, REAXYS, REAXYS_BIOCATALYSIS database, our tool offers high-accuracy predictions, reflecting the latest in chemical research and data.
Strategy Settings
Precursor scoring | Relevance Heuristic |
---|---|
Min. plausibility | 0.01 |
Model | Template_relevance |
Template Set | Pistachio/Bkms_metabolic/Pistachio_ringbreaker/Reaxys/Reaxys_biocatalysis |
Top-N result to add to graph | 6 |
Feasible Synthetic Routes
Disclaimer and Information on In-Vitro Research Products
Please be aware that all articles and product information presented on BenchChem are intended solely for informational purposes. The products available for purchase on BenchChem are specifically designed for in-vitro studies, which are conducted outside of living organisms. In-vitro studies, derived from the Latin term "in glass," involve experiments performed in controlled laboratory settings using cells or tissues. It is important to note that these products are not categorized as medicines or drugs, and they have not received approval from the FDA for the prevention, treatment, or cure of any medical condition, ailment, or disease. We must emphasize that any form of bodily introduction of these products into humans or animals is strictly prohibited by law. It is essential to adhere to these guidelines to ensure compliance with legal and ethical standards in research and experimentation.